As my final post in what has become a three-part series on nuclear energy, I thought I would talk about specific famous nuclear accidents and what they can tell us about nuclear power. Because it can get technical, I’ll provide links to more detailed resources. My goal is to show that these accidents were not the inevitable consequences of an inherently dangerous technology, but that they were like other severe industrial disasters, caused by poor planning or irresponsible management.
Famous Meltdowns
If you haven’t read the previous entry, I would advise you to do so.
When you picture an accident at a nuclear power plant, you’re likely thinking of a meltdown. This is when the fuel assemblies get so hot that they damage themselves or parts of the reactor, possibly releasing radioactive material. A bad enough meltdown will cause this fuel to literally melt, creating a kind of lava made from the molten fuel assemblies, reactor parts, and any concrete and steel nearby. This material, dubbed corium by scientists, is extremely radioactive and continually heats itself with radioactive decay, just like spent fuel rods we discussed last time, but now lava. Unless kept cool, corium can melt through concrete and potentially escape the reactor. To date, there are three instances of nuclear power plants suffering these kinds of meltdowns, and I imagine you’ve heard of them.
Three Mile Island
On 28th of March 1979, one of the two reactors at the Three Mile Island Nuclear Generating Station in Pennsylvania, USA suffered a partial meltdown. The station used pressurized water reactors, where water at 15 megapascals (150 times normal pressure) acts as the moderator and coolant for the fuel. Pressurized liquids boil at higher temperatures, so the water heats up to almost 300°C without boiling. This superheated water is pumped through heat exchangers to transfer its heat to non-pressurized water, which boils into steam to power turbines. Hours before the meltdown, an accident while cleaning a filter caused one of the pumps that circulate the pressurized water to stop working. This pump was repaired, but not before this temporary loss of cooling caused water to expand and a pressure relief valve to open. The valve was not designed to automatically close itself and a poorly designed instrument panel meant that no one realized the valve was open, so the cooling system was no longer airtight and water pressure started dropping. Technicians could tell that something was wrong and shut down the reactor, but it still required constant circulation of coolant due to leftover heat. Due to more problems with control room design, technicians weren’t able to figure out what exactly was wrong before the pressure dropped so low that cooling water started to boil and the fuel rods started to melt. This video from the NRC provides a deeper explanation.
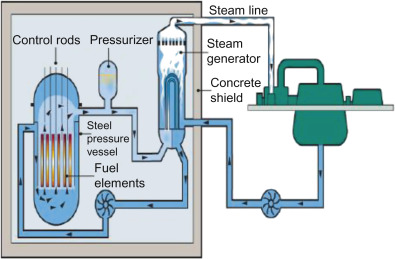
Fortunately, Three Mile Island was not as severe as it could have been. Coolant circulation was re-established quickly, preventing a complete meltdown. The corium that was generated didn’t escape the reactor. While radioactive gas was released into the air, the amount of radiation absorbed by those living near the plant was only 14 microsieverts, less than that of a chest x-ray. To date, no deaths have been definitively linked to the disaster and there has been no statistically significant increase in cancer or other radiation-related illnesses. Like all nuclear plants, TMI was built to contain the effects of a meltdown, and it absolutely succeeded in this regard.
So much of the reason why the Three Mile Island accident is remembered so poorly is because of failures to communicate with the public. Both the plant and the Nuclear Regulatory Commission put too little effort into explaining the situation to news media, leading to inaccurate reporting of the problem. Politicians weren’t kept informed, leading to contradictory decisions and messaging to the public. A good example of this was how two days after the meltdown, there were concerns about the probability that the reactor could explode due to hydrogen gas building up inside the reactor. This was shared with the public and hasty evacuations were ordered, but contradictory messages were sent as scientists weren’t certain what the risk of an explosion was and all of this debate was reported unfiltered. Within two days, it was determined that an explosion was not possible, but this fact was not shared with the public for over a day.
Several regulatory changes were made to how controls worked and technicians were trained in American nuclear power plants, and modern system automation and computer displays would prevent this specific accident. But the plant was designed to contain a meltdown and in that it succeeded. To learn more, I strongly recommend this video from Kyle Hill’s Half-life Histories series.
Fukushima Daiichi
On 11th of March 2011, the Tohoku earthquake and resulting tsunami caused three of the six reactors at the Fukushima Daiichi Nuclear Power Plant in Fukushima, Japan to meltdown over the course of three days. The plant used boiling water reactors, where the nuclear fuel directly boiled water to create steam to turn turbines. When the earthquake occurred (the fourth largest on record), all active reactors were shut down and diesel generators were switched on to keep cooling water circulating. But when the tsunami hit, it flooded these generators and shut them down. With no way to keep water circulating, the three active reactors slowly melted down. Similar to Three Mile Island, hydrogen gas was released from chemical reactions and built up within the reactor. Unlike TMI, this hydrogen was able to leak out of the reactor vessel, build up inside the containment building, and cause explosions in two of these three buildings. Radioactive material was spread to the surrounding environment by these explosions and by seawater that had been used to keep the reactors cool as a last ditch effort. This video from the IRSN provides a deeper explanation.
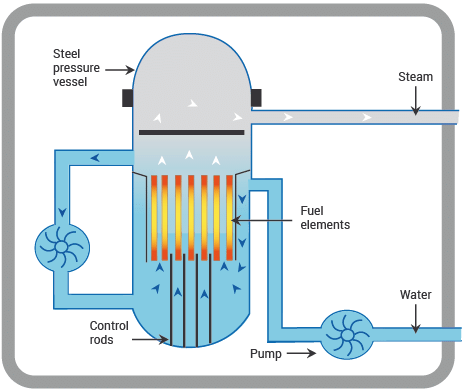
In terms of people evacuated, Fukushima was the second largest nuclear disaster in history at 81,000 evacuees. But this was because the population density around the power plant was so high. The highest radiation dosage detected in surrounding communities was about 30 millisieverts a year. Compare this to the roughly 4 millisieverts a year that one would be exposed to normally and to the 50 millisieverts a year that the EPA sets as the maximum yearly dosage radiation workers are allowed to receive. To date, no deaths have been linked directly to radiation, though much of this has to do with the timely evacuation. An exclusion zone covering 807 square kilometers (312 square miles) was declared, requiring everyone within this zone to evacuate. But today, due to radiation subsiding naturally and extensive decontamination efforts, this exclusion zone has shrunk to 371 square kilometers (143 square miles). While corium managed to melt its way through and escape all three reactors, all this corium was contained to the bunker-like containment buildings.
Though Fukushima was a catastrophe, the disaster that caused it was a once-in-a-millenium disaster in its own right. That said, it would be wrong to say it was unpreventable. For decades before the Tohoku earthquake, scientists and regulators had raised concerns about the plant’s lack of sufficient tsunami protection. TEPCO, the utility company that owned the Fukushima-Daiichi power plant, has a history of false reporting in regards to safety inspections and lobbying to prevent enforcement of safety regulations. The Onagawa Nuclear Power Plant, which wasn’t operated by TEPCO, was located closer to the earthquake’s epicenter and experienced a higher tsunami. But the Onagawa plant was deliberately built at a 5 meter higher elevation with a 5 meter higher seawall, so it suffered no major flooding or damage to the reactors and was even used as a shelter for displaced locals. This video, also from the Half-life Histories series, provides more information. Nuclear agencies around the world have developed plans to harden nuclear power plants against natural disasters, but Onagawa shows that even disasters like the Tohoku earthquake can be weathered with a sufficient dedication to safety in construction and regulation.
Chernobyl
On 26th of April 1986, one of the four reactors at the Chernobyl Nuclear Power Plant in Ukrainian SSR, USSR suffered a catastrophic meltdown. The plant used RBMK reactors, a Soviet design consisting of a massive cylinder of graphite with vertical channels running through it. Suspended in these channels were fuel and control rods while water was pumped through these channels to be boiled into steam by the nuclear reaction. The day before the disaster, the reactor was planned to be shut down as part of a safety test, but this test was delayed due to the surrounding community’s electricity needs. This delay meant two things; the test would be performed by the less-experienced night shift, and the reactor was shut down too fast. For reasons I will gloss over, nuclear reactors needed to be shut down slowly, over the course of several hours. By shutting down too fast, waste products built up in the fuel rods which made it harder to start the reactor back up. The normal procedure to fix this would be to slowly nurse the reactor back to full power over the course of days, but the operators were impatient. In a profound act of poor management and in violation of reactor protocols, the reactor was cranked up to full power by pulling almost all of its control rods all the way out in order to get the reactor working quickly.

I briefly touched on moderators last time; materials that, when placed between the fuel rods, make nuclear chain reactions easier. Because hydrogen is a good neutron moderator, most reactors today use water as their moderator. Water is inexpensive, it can double as a steam source, coolant, and radiation shield, and it can make reactors much safer by creating a negative feedback loop. If the reactor gets too hot, steam bubbles will form between the fuel rods, meaning there’s less moderator in the reactor. The hotter the reactor gets, the harder it is for it to get even hotter. The one big disadvantage of water as a moderator is that the most common isotope of hydrogen (1 proton, 0 neutrons, 99.98% of hydrogen atoms) only acts as a moderator for more enriched fuel*, making these reactors more expensive to run. For budget reasons, Chernobyl only used lowly-enriched fuel, so regular water would actually prevent more fissions than it caused. Because of this, the earliest nuclear reactors and the RBMK reactor used carbon in the form of graphite as a moderator. Graphite is inexpensive to acquire and works for low-enriched and unenriched uranium. The major downside of graphite (and this is a big one) is that it doesn’t have the negative feedback loop of water; the hotter the reactor gets, the easier it is to get even hotter with graphite. At the time, the RBMK reactor was the only reactor design on Earth that didn’t have this basic safety mechanism.
Another weakness of graphite as a moderator is that it’s a solid; when the control rods are pulled out, you’re left with an empty pipe with no moderator in it. To remedy this, a second rod made of graphite was attached to the bottom of each boron control rod; when the control rod was pulled out, more moderator was pulled in. With these graphite “tips” making fissions more likely, the reactor quickly exceeded their maximum safe temperature. To prevent a meltdown, the staff reinserted all the control rods at once to kill the reaction. As the control rods were reinserted, the graphite tips had to pass between the bottom parts of the fuel rods. Due to how neutrons move through such reactors, this concentrated the immense amount of neutrons generated by the overheating reactor onto the bottommost part of the fuel rods. In an already overheating reactor, they’re rate of fission skyrocketed.
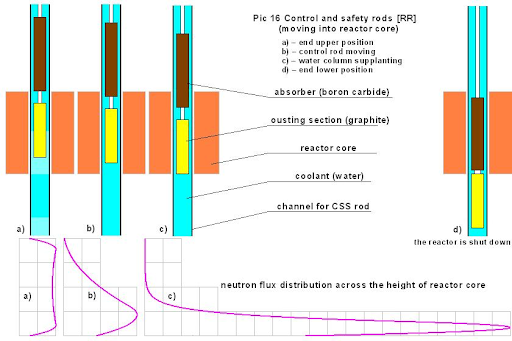
What happened next is still debated, but the leading hypothesis is that the fuel rods flashed hot enough to split water molecules into hydrogen and oxygen. Expanding gasses blew the lid off the reactor, allowing oxygen to rush in and ignite this hydrogen. The resulting explosion tore open the reactor and the building while igniting the graphite. The fuel rods melted into corium and spilled into the basement while the graphite fire buoyed radioactive particles into the atmosphere. This video from Hank Green provides a deeper explanation. (I glossed over a lot of technical material, so I would recommend this video for a more complete picture.)
The Chernobyl disaster is by far the worst nuclear accident in history. It released over ten times as much radiation as the Fukushima meltdown. The exclusion zone from the disaster is 2,600 square kilometers (1,000 square miles) and required the evacuation of 350,000 people. 31 people died from the meltdown’s immediate effects, namely plant workers from the explosion itself and first responders from radiation poisoning. The death toll from released radiation is hard to determine due to the impossibility of determining the exact cause of any one case of cancer or disease, but statistical models predict somewhere between 4,000 and 14,000 cancer deaths would not have occurred if the meltdown didn’t happen. Some residents of the nearby town of Pripyat received 100 millisieverts of radiation, just enough to measurably increase one’s risk of contracting cancer, and while radiation levels have waned significantly in the 35 years since the disaster, there are still parts of Pripyat giving off between 8 and 80 microsieverts a year.
The meltdowns at Three Mile Island and Fukushima both occurred in reactors that had already been shut down, with the heat that caused the meltdowns coming from leftover radioactive decay. The Chernobyl meltdown occurred in a reactor that was actively fissioning and at least ten times hotter than its maximum safe energy output. While the Fukushima reactor buildings were damaged by hydrogen explosions, the flammable hydrogen gas had already leaked out of the reactor vessels, away from the most radioactive parts of the reactor. When the Chernobyl reactor exploded, it originated from inside the core, launching the most radioactive material from the reactor into the surrounding environment. Like all nuclear reactors in the developed world, each reactor at Three Mile Island and Fukushima was built inside its own containment building, a heavy bunker of concrete and steel designed to contain as much radioactive material as possible in the event of a meltdown. Due to budget constraints, Chernobyl had no such specialized containment building. Today, only 8 of the 26 RBMK reactors ever built remain in use, all of which have been modified to fix the design flaws that killed Chernobyl. The RBMK design would never have been approved outside of the Soviet bloc and no reactor design in use in the developed world could fail this badly.
Takeaway
All three of these meltdowns have significantly contributed to public fear of nuclear energy. While it is true that nuclear fission and its by-products are dangerous to human life and should be treated with the utmost respect, the same could be said of numerous industrial processes that we take for granted. Uranium compounds are one of 840 entries in OSHA’s database of regulated occupational chemicals. This Wikipedia list of industrial disasters (hardly a comprehensive list obviously, but good for a sample size) lists 36 major accidents in the energy sector since 1957, and only four of them are nuclear accidents. (The three here are included) The worst disaster on this list in terms of loss-of-life is the 1975 Banqiao dam failure in Henan, China, causing over 250,000 deaths in total. On top of the Fukushima meltdown, it also lists two other disasters caused by the Tohoku earthquake and tsunami; a dam failure in Shukugawa and a natural gas tank fire in Chiba. This is not to say that these types of disasters are inherently greater cause for concern than a nuclear accident, there are aspects of nuclear disasters that are uniquely dangerous. But these are all risks that we choose to manage because of the benefits that come from having this infrastructure. And in all of these cases, the solution to mitigating disasters are the same; well-designed infrastructure and safety regulations that protect workers and surrounding populations while ensuring dangerous waste is either made safe or disposed of where it can no longer cause harm. Nuclear industry is no different and is in many ways much safer, as I covered last time. There are inherent risks that must be carefully managed, just like with everything else.
For More Details
*For this reason, not all water-moderated reactors use regular water. Some reactors use heavy water, water who’s hydrogen is the less common Hydrogen-2 isotope (1 proton, 1 neutron). These reactors can use less enriched fuel, but are also expensive to run because heavy water is hard to produce. Only 0.02% of all water molecules are heavy water and producing enough heavy water for nuclear reactors requires filtering these molecules out of regular water in a long and energy intensive process.
Comments