Stem Cell Therapy
- WSP Rhodes
- Oct 27, 2024
- 13 min read
There were some recent stories in the news about a study reversing a patient’s type 1 diabetes using stem cell therapy. These are very promising results with the potential to lead to a cure once further research is done, but I wanted to use this occasion to talk about stem cell therapy. Stem cells have been a topic of public discourse for a few decades now, with both controversy and overpromising obscuring the actual science. So without further ado…
What are Stem Cells?
In order to make multicellular organisms work, the 36 trillion cells in the human body need to be highly specialized. There are roughly 200 types of cells in the human body, each with different shapes, sizes, internal and external structures, and expressed proteins to facilitate their unique function. But each of these cells, regardless of their specialization, has the exact same genome with the exact same instructions to turn a cell into any of the 200 specializations. What differentiates a nerve cell from a blood cell from a muscle cell is the regulation of these genes; the genes associated with their specialization are activated while the genes associated with every other specialization are permanently switched off. Stem cells are cells that have not fully specialized; they haven’t grown any of the specialized structures associated with differentiated cells and don’t express any specialized proteins. All multicellular organisms start out as a bundle of completely undifferentiated cells, called pluripotent* stem cells, which theoretically could differentiate into any cell type. But stem cells don’t have to be completely-undifferentiated pluripotent cells, they can also be partially-differentiated multipotent cells. These stem cells have begun to differentiate, having switched on some differentiated genes and permanently switched off others, but still haven’t chosen their final form. A good example of multipotent cells are hematopoietic stem cells, the cells in the bone marrow from which all blood comes from. These cells constantly reproduce with half of each generation differentiating into one of twenty different cell types, including red blood cells, platelets, and the various types of immune cells, but they can’t turn into other cell types like bone cells. You can picture the different types of stem cells as something like a family tree, with pluripotent stem cells at the trunk, differentiated body cells at the brand tips, and the different levels of differentiated multipotent cells between them.
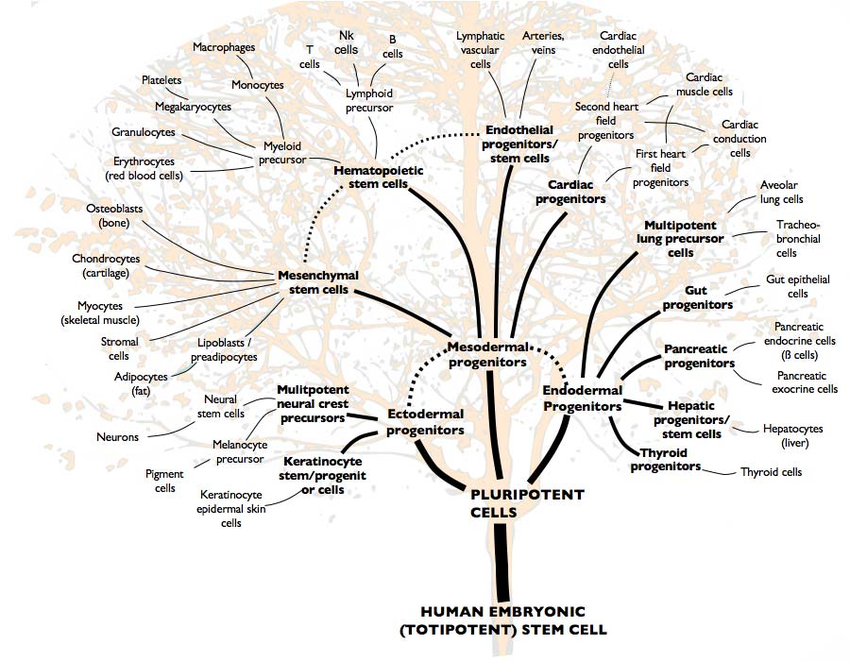
To give some perspective as to how this differentiation process works, I’ll talk briefly about embryonic development. After conception, the single cell that’s created divides into a ball of identical, undifferentiated cells (called a blastomere). Once this ball is roughly 70 cells strong, cells begin to differentiate. Receptors on the membrane of each cell determine each cell’s position relative to each other; i.e. are they on the surface of the ball or in the interior. Depending on the answer, these receptors trigger one of two cascades of signaling proteins which each end with a specific group of transcription factors binding to the cell’s DNA at specific locations (see previous post on cell signaling). These transcription factors switch on certain genes necessary for the cell’s chosen role while permanently switching off genes necessary for other roles. The end result is that the outer layer of this ball becomes placental precursor cells, cells that develop into the placenta once the zygote implants in the uterine wall, while the cells inside the ball become pluripotent cells that become the embryo itself. Among the genes activated in these pluripotent cells are instructions on how cells should grow or move, so these cells contract into each other to form a disc inside this sphere of placental cells. Skipping over a lot of specifics, this disc differentiates into three distinct layers; the top layer becoming ectodermal cells (which will eventually become skin and nerve cells), the middle layer becoming mesodermal cells (which will eventually become muscle, bone, vascular, and blood cells), and the bottom layer becoming endodermal cells (which will eventually become intestinal, liver, and lung cells). All these cells continue to follow instructions laid out in their DNA for how to behave given their location; for example, the ectodermal cells that are located along the discs centerline will fold in on themselves to create a crease in this ectodermal layer. This crease pinches itself off from the ectodermal layer to create a tube of ectoderm cells beneath the main layer. These cells in this tube differentiate into nerve cell progenitors to eventually become the central nervous system while the main ectodermal layer becomes skin cell progenitors. Processes similar to this occur throughout the embryo until all body structures have been created and almost all cells are differentiated.
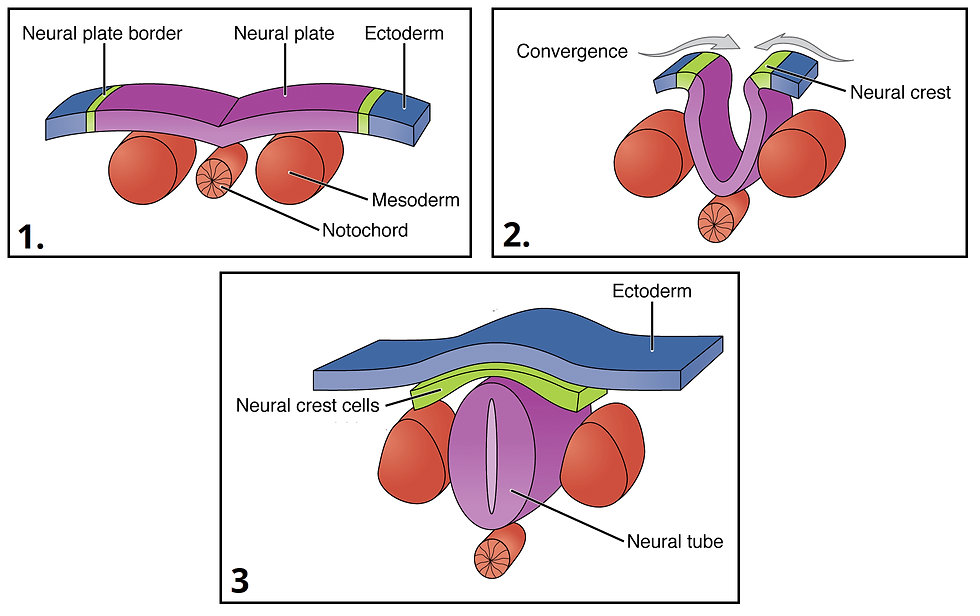
Formation of neural tube; the progenator of the central nervous system.
By the time we leave the womb, there are no pluripotent cells left in the body. But there are still a few cells in the body that aren’t fully differentiated. I’ve already mentioned your bone marrow, which contains hematopoietic stem cells that become your blood, but also contains mesenchymal stem cells that can become bone, muscle, or connective tissue. Tissues that grow continuously, including skin, hair, and the lining of the stomach and intestine, do so by having multipotent stem cells at their bases that continually produce new cells. There’s strong evidence to suggest that the stem cells which produce new neurons continue to live in remote parts of the brain throughout one’s life, allowing for continued neurogenesis even after childhood. Most differentiated cells have a limited number of times they can reproduce (see previous post on aging), but stem cells can reproduce continuously. It is this ability to continuously reproduce and to take the place of any body cell that makes stem cells a continued topic of research.
Potential Therapeutic Uses
Stem cell research as we would describe it today began in 1981, when the first stem cell lines were developed for research purposes. Some of this research involves producing tissues for lab use, such as observing how cells develop or testing drugs on lab-grown tissues. But the most notable research goal has been the use of stem cells in regenerative medicine, adding new cells to a damaged organ to replace damaged cells and repair damaged tissue. Illnesses with the potential to be treated in this way include neurodegenerative diseases such as Alzheimer’s, Parkinson’s, and ALS, spinal cord injuries, traumatic brain injuries, several forms of heart disease, certain forms of blindness or deafness, and diabetes. Stem cells could also improve musculoskeletal disorders and wound healing. Pluripotent stem cells would also be a necessary component of bioprinting and tissue engineering, letting one grow or build new organs to replace defective ones (see here for more details). Anywhere that medical treatment is hampered by the limitations of human tissue healing, there is potential for stem cell therapy to significantly improve health outcomes.
But of course, there is still work to be done before this research actually turns into treatments. The biggest area of research is fully understanding and controlling the mechanisms of differentiation. If you inject a muscular dystrophy patient with stem cells, you’ll want to make sure those stem cells turn into muscle cells. You’ll also want to make sure they become muscle cells in the exact parts of the body that need muscle cells, and that they reproduce into the exact number of muscle cells you want (too few and they won’t treat the disease, too many and they could create tumorous growths). Pouring clay on a building site doesn’t guarantee they’ll turn into bricks, much less assemble themselves into a house. Now, there is evidence that the presence of stem cells in damaged tissue does promote tissue repair, as they secrete signal proteins that reduce inflammation, recruit repair cells, and reduce scarring, so there is some healing occurring other than just replacing damaged cells. But the development of malignant tumors is an observed risk for lab animals injected with stem cells. Remember, the reason most body cells can’t reproduce indefinitely is as a safeguard against cancer, so cells that can reproduce indefinitely are more prone to becoming cancerous. Another big problem is tissue rejection; if you inject a patient with stem cells from a donor, their immune system will likely recognize the cells as foreign and attack. This can be prevented by using stem cells from the patient’s own body, but that raises the problem of finding and/or creating them. Since 2006, scientists have been able to turn differentiated body cells into pluripotent stem cells via genetic manipulation. But finding ‘reprogramming’ strategies for differentiated cells that are efficient and produce cells that are healthy, non-tumorigenic, and viable for therapy compared to non-induced embryonic stem cells has been a challenge.
This does bring us to the ethical considerations that you might be most familiar with when discussing stem cell therapy. Since human stem cells were first isolated and cultured in 1998, the best source for them have been human embryos with the process of collecting them necessarily destroying the embryo. This has led to multiple countries banning research using embryonic stem cell lines and strict ethical guidelines being placed on research and funding. Now, it’s important to understand that the majority of research is done on embryonic stem cell lines; because stem cells can reproduce indefinitely, it is possible for scientists to ‘farm’ stem cells indefinitely. All embryonic stem cell research done today is done using these cell lines, done on cells whose ancestors were taken from a human embryo but have been grown in lab cultures for thousands of generations. It should also be noted that these cell lines aren’t created from aborted fetal tissue; pluripotent stem cells can only be collected in the first few weeks of embryonic development. The principal source of stem cells for research and cell lines are leftover blastomeres created for IVF treatments that weren’t implanted. And as mentioned earlier, embryonic stem cells carry a risk of tissue rejection since they aren’t genetically identical to the recipient, so there is an incentive for doctors to use non-embryonic induced stem cells for any potential stem cell treatments.
On the other end of the spectrum, we have commercial stem cell clinics. These are for-profit businesses that claim to treat a wide range of diseases using stem cell therapy. The therapies they peddle do not go through clinical trials and do not have regulatory approval. They claim to be able to treat a myriad of minor and unrelated ailments with identical therapeutic methods, from diabetes to knee pain. They charge exorbitant fees for these treatments and there are frequent cases of serious injury caused by side effects, not to mention few if any successes. Stem cell therapy has been promised to be a magic bullet of medicine, which is a claim bound to attract charlatans. But the existence of these “clinics” has unfortunately soured public trust in what is a promising field of medical research. I’d recommend this resource by EuroGCT if you are interested in the difference between trustworthy and untrustworthy stem cell medicine, as well as my previous post on identifying misleading information. But legitimate research is being done on stem cell therapy, even if it’s sometimes obscured by misinformation.
Present and Future
To date, the only form of stem cell therapy that is widely used is hematopoietic stem cell transplantation, more commonly known as a bone marrow transplant**. This is done to replace the blood-producing stem cells lost during cancer treatment or to treat genetic disorders that affect the blood or the immune system. For cancer patients, stem cells can be extracted and saved before treatment so as to be reintroduced to the body after treatment, though occasionally a separate donor’s stem cells is needed. For treating genetic disease, the patient’s existing stem cells must be completely killed off via drugs or radiation before being replaced by cells from a donor, usually a close family member. This leaves the patient with blood and an immune system genetically identical to the donor, specifically chosen to not have the disease in question. Transplants from a donor carry similar risks of tissue rejection as organ transplants, made worse by the fact that the patient has a donated immune system that would perceive the rest of their body as foreign. But as we’ve discussed before, there’s already work being done on genetically engineering hematopoietic stem cells to remove genetic diseases, allowing one to treat these diseases with self-transplants. There’s also recent research into using hematopoietic stem cell transplants to treat extremely severe autoimmune diseases such as multiple sclerosis, essentially by killing off and replacing the patient’s immune system to erase their immune memory. Hematopoietic stem cell transplantation remains a dangerous procedure with a lot of risk for complications, hence why doctors don’t use it to treat mild autoimmune diseases or allergies, but it is a treatment method for multiple disorders and ongoing research to refine the technique.
An example of ongoing research is the one I mentioned at the beginning; using stem cells to effectively cure type 1 diabetes. Type 1 diabetes mellitus is an autoimmune disorder where the immune system mistakenly recognizes a patient’s insulin-producing beta cells as a threat. These cells can normally only be produced by other beta cells reproducing, so killing off all the beta cells in the body results in their extinction within the patient and the permanent loss of insulin production***. Transplanting new beta cells into a diabetic patient would in theory correct this, but donors are very hard to come by and tissue rejection would be a concern. The study I mentioned earlier involved taking cells from a participant with type 1 diabetes, reverting them into pluripotent stem cells, turning them into beta cells, and reimplanting them in subject’s abdominal wall (the location ensures the cells can be monitored and serviced more easily than if they were in the pancreas). Two months post-procedure, the subject reported no longer needing insulin injections, and has remained so for over a year. These same researchers have performed this procedure on type 2 diabetic patients (who have fewer but still some beta cells), but this is the first time stem cell-derived beta cells have been used to replace completely lost insulin production. Now, this subject was already on immunosuppressants for a previous liver transplant, so the risk of her immune system killing off these beta cells was mitigated in a way that wouldn’t be true of most patients. Finding a way to prevent the autoimmune response to the new beta cells is the next step for researchers. Fortunately, the ability to create beta cells using stem cells does make it easier to research the process of their destruction by the immune system. Researching how the immune system attacks beta cells has been difficult in the past because by the time a patient is diagnosed with diabetes, the patient’s beta cells are already gone and there’s nothing left to observe. The ability to create specialized cells on demand allows for studies using animal and in vitro models to observe these attacks, which could lead to ways of defending the newly transplanted beta cells. This could take the form of genetically modifying beta cells to not express proteins that activate immune responses or implanting the beta cells in a container that immune cells can’t get inside. There is still a way to go before these studies turn into a permanent treatment for diabetes, but it is a big step in the right direction.
Finally, I’d like to talk about a very new area of research; that of stem cell exosomes. Exosomes are small bubbles of cell membrane (hundreds of times smaller than a cell) that are secreted by cells and contain proteins, mRNAs, and other molecules from said cells. These vesicles can travel through the body and can be absorbed by other cells, moving their contents into the receiving cell. Exosomes are an emerging area of research, but they’ve already been discovered to be an important means of communication and transport between cells with numerous impacts on health and potentials for treatment. Remember, mRNAs are the instructions cells use to build proteins, and these proteins can be transcription factors which affect how a cell behaves. Exosomes allow one cell to transfer instructions directly to another cell and impact how the receiving cell behaves. There’s evidence that immune cells use exosomes to selectively activate T-cells to target a particular attacker. Cancer cells have been found to produce exosomes to inhibit immune responses and to control surrounding tissues, just as healthy cells have been found to produce exosomes that keep cancer cells behaving normally. Scientists are looking into using exosomes as diagnostic markers (collect exosomes from a patient’s blood or urine to see what their cells are talking about) and potentially as drugs or as delivery mechanisms for drugs. I bring exosomes up here because I mentioned earlier that the mere presence of stem cells in damaged tissue can induce healing. There is considerable evidence that the reason for this are exosomes being produced by the stem cells that contain materials and instructions to make adult, differentiated cells behave slightly more like stem cells, regenerating tissue without scarring. This has led to research into using stem cell exosomes for therapeutic purposes in place of the stem cells themselves, increasing the volume of the active agent while giving all the benefits of stem cells with none of the drawbacks. Now, this is a very new field of study; we are not yet at a place where we can use exosomes for medicinal purposes. We’re still figuring out what effects the specific mRNAs and molecules in an exosome have on the receiving cell, and the way we manufacture exosomes currently is to culture cells and filter the exosomes out of the growth medium, which is inefficient and doesn’t produce exosomes of consistent quality. But this is an extremely promising field of study with applications inside and outside of stem cell research.
Stem cells have been a field of research for several decades now, all the while making breathtaking promises of what they can be used for. And these promises aren’t hollow; the research done on stem cells tells us about the internal mechanisms of cells and tissues, knowledge we’ll need if we are to hack these processes to our benefit. It is taking a long time for these promises to come to pass, as it takes a long time to understand something well enough to control it. And this research has been marred with ethical concerns, some based on misinformation, some not. But a lot of modern medical research involves using living cells or the products of them for therapeutic purposes, from using genetically modified T-cells to kill cancer to using extracellular matrix to treat chronic wounds. Stem cell therapy folds very neatly into this new school of treatment and the research done on stem cells has allowed a lot of it to exist. There’s so much research going on behind the scenes, and it’s slowly starting to lead to treatments.
For More Details
*Technically, pluripotent stem cells are not the first or most undifferentiated cell type to form. When a sperm and egg first meet to form a zygote, it is made up of totipotent stem cells. Totipotent stem cells can then differentiate into pluripotent stem cells that eventually become the body or cells that eventually become the placenta. For simplicity’s sake however, we’ll call pluripotent cells the most undifferentiated cell type.
**I’m using the common name for simplicity’s sake, but I should say that the term is technically inaccurate. Bone marrow consists of more than just hematopoietic stem cells, such as other types of stem cells, fat cells, and connective tissue. Only the hematopoietic stem cells are actually transplanted during these procedures, after being extracted from the rest of the bone marrow, and it is possible to extract these stem cells from other sources, such as filtering them out of the donor’s blood or from amniotic fluid.
***Shortest possible explanation of insulin; your body wants to maintain a very careful balance of sugar in the blood. Too little sugar and cells starve, but too high and cells have trouble storing it. To keep blood sugar in a goldilocks zone, the pancreas monitors blood sugar and secretes two hormones to raise or lower it. Insulin is secreted when blood sugar is too high, telling liver and fat cells to convert excess sugar into an unusable form called glycogen. Glucagon is secreted when blood sugar is too low, telling liver cells to convert glycogen back into usable sugar. How chronic high blood sugar causes health problems is complex and multifaceted, but a major mechanism is that it causes blood vessels to become rigid and narrower, reducing circulation.
Comments