The word CRISPR has become more common in scientific circles in the past few years. It’s a revolutionary new technology with incredible potential for the field of genetics. But similar to the debut of other revolutionary technologies, many have pointed out how CRISPR could be a Pandora’s box of potential dangers. So I thought it would be a good idea to make sure everyone understands what this technology actually is, since the term sometimes gets thrown around without being fully explained. This will hopefully allow us all to understand what the benefits and threats created by this technology actually are and to finally allow me to talk about science ethics in one of these posts. So without further ado…
What is CRISPR?
It would be useful to reread my previous post about bacteria and viruses as these concepts are central to understanding CRISPR.
Just like us, bacteria can be infected with viruses. In fact, because bacteria cells are far simpler than ours, it is far, far easier for bacteria-infecting viruses (called bacteriophages or phages) to do so. Every day, 40% of all bacteria in the world’s oceans are killed by bacteriophages, though they reproduce fast enough to replenish their numbers. (Fun fact, phages can’t harm eukaryotes like us, so there is research being done into using phages to fight bacterial infections in humans, as this video from Kurzgesagt describes.) Bacteriophages are built like syringes with grasping legs (if you’ve ever seen a virus depicted as a weird spider thing, that was a phage) which grab hold of bacteria and inject their genetic material directly through the cell membrane. Obeying the viral DNA’s instructions just as it would its own, the bacteria cranks out thousands of identical phages before ripping itself open to release these new phages on other bacteria.

So just like multicellular life, half of all bacteria species have been found to have a sort of acquired immune system to combat viral infection. On the off-chance that a bacteria is infected but manages to identify the viral DNA as an invader, it will cut this DNA into pieces and incorporate these pieces into its own genome. These equal-sized chunks of viral DNA are inserted into a special region of the bacterial genome, with segments of DNA between them so the viral instructions don’t accidentally get read. It’s from these in-between segments (called repeats) that the name CRISPR comes from, short for Clustered Regularly Interspaced Short Palindromic Repeats. When sequencing this region of the bacterial genome, one would see a pattern of longer, unique segments of viral DNA (called spacers) alternating with shorter segments that are all identical to each other, spaced apart evenly. The spacers contain a record of every enemy the bacteria has faced, which it can use to protect itself from said enemy again.
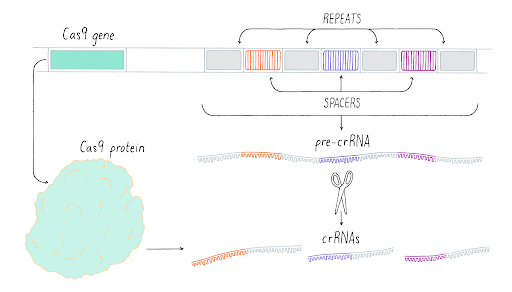
Once this viral DNA has been incorporated into this permanent record, it can be used to prevent any further infection by the same virus. RNA copies are made of each spacer which are then attached to a protein called Cas9. Using this segment of RNA as a template, the Cas9 protein attaches itself to any strand of DNA that matches this template and cuts it in half. If viral DNA from the same species of phage ever enters the bacterial cell again, Cas9 can effectively destroy it before it poses a threat. And Cas9 has extremely high specificity, meaning it always targets DNA that matches its template and rarely-if-ever targets DNA that doesn’t match, even if it is very similar. Much like the adaptive immune system in humans, this makes the bacteria effectively immune to any virus that it has been infected with before.
Genetic Engineering
Genetic engineering is a process wherein the genome of an organism is directly altered using biotechnology. This involves making a cut in a cell’s DNA on a very specific gene, in order to disable this gene or to create space to insert a new gene. For example, the Flvr Savr tomato, the first genetically engineered organism made for human consumption, had a new gene added that interfered with the production of enzymes which contributed to ripening, creating a line of tomatoes that aged far slower. Historically, one of the hardest parts of genetic engineering was making this first cut in exactly the right spot. In nature, there are proteins called restriction enzymes which make cuts to very specific sequences of DNA called restriction sites. So the earliest cases of genetic engineering, including creating the Flvr Savr, were done using human-made restriction enzymes. But creating an enzyme which cuts the precise gene you want to cut is extremely difficult. The exact intermolecular dynamics which allow proteins to “talk” to DNA is still poorly understood (protein intermolecular forces aren’t well understood in general), so new lines of these enzymes could only be made by trial and error, mutating existing restriction enzymes until a mutant proved it targeted the right gene. Ensuring that new transgenes are inserted into the correct part of the genome is exceptionally important due to how a gene’s location can influence how it and other genes are used. In 2002, a study in Paris used a form of gene therapy to treat SCID (the disease the bubble boy had) in 15 infants. While the treatment was largely effective, four were later diagnosed with leukemia or similar illnesses. The new gene had been accidentally inserted right next to an important anti-cancer gene, which made this gene less effective.
What would radically improve genetic engineering technology would be a molecule that can make these same cuts in DNA, but was more versatile. Something that was essentially programmable, easily retargeted toward a different gene. And something with extremely high specificity, that always targeted the right genes and never the wrong genes.
In 2012, research scientists Jennifer Doudna and Emanuelle Charpentier published their findings that Cas9 proteins could be used as a programmable gene editor, drastically reducing the time and cost needed to genetically modify an organism. Today, this is considered one of the most important discoveries in the field of biology. Genetically modifying cells has become something that most labs can do, which has numerous potential practical applications;
Treating genetic diseases by cutting out and replacing genes that cause disease. This is still in the research stage but is very promising.
Treating cancer. Studies have been done where T-cells were removed from a cancer patient, modified with Cas9 to make them aggressively attack cancer cells, and released back into the patient. Results so far have been promising.
Eliminating persistent viral infections such as HIV. Infected cells treated with CRISPR-Cas9 were shown to have this viral DNA removed.
Biosynthesis of medicine and other biological agents. The first GM organisms to be used by the public was in 1978 when E. coli bacteria were modified to produce human insulin. Almost all insulin for diabetics for the past 40 years has come from these bacteria.
Improved experiments and biotechnology. Many DNA testing systems, both for laboratory and public use, make use of small bits of DNA that attach to specific genes. Many experiments require specific strains of bacteria, fungai, or even animals that have been modified in a precise way for specific experiments. Anything that makes it easier to edit DNA will make these experiments cheaper and easier to perform.
Making genetic engineering of organisms easier has applications for agriculture and biofuel production.
And these are just the applications that are currently being experimented with. As more research is done, more avenues for research will be discovered.
Ethics
I’m certain that for most of you, any discussion of genetic engineering and GMOs is accompanied by a certain degree of fear and skepticism. A lot of this is baseless, particularly around the safety of GMO food and other products. But there are genuine problematic elements around this technology, and it’s important to understand exactly why they are problems.
I could probably do a whole post about the myths and misconceptions around GMO food, but the short answer is that GMO food is as safe to eat as non-GMO food. It’s usually only one gene that is changed in each modified crop line, which isn’t enough to make it unsafe to eat. For comparison, every major plant and animal that we eat has been selectively bred for thousands of years and many are no longer recognizable as their original species. (One of my favorite facts; cabbage, kale, broccoli, cauliflower, and brussel sprouts are all the same species, different breeds of wild mustard.) And breeding like this changes far more of the genome in a far less controlled way than genetic modification. (Another fun fact; by breeding roses for their appearance, breeders accidentally reduced the fragrance of many breeds, just because they had no control over secondary effects.) Also, any GMO that’s sold to the public, especially for human consumption, is rigorously tested for safety and monitored, so any potential health problems are discovered early. Ultimately, nutrition is not the threat posed by GMO crops.
The threat that GMO crops could pose to society is one of economics and copyright. The companies that create strains of GMO crops regularly claim legal ownership of their creations, including the progeny of said plants. In 2009, the company Monsanto successfully argued before the US Supreme Court that farmers who had Monsanto corn accidentally growing on their land due to natural spread of seeds from other nearby farms were guilty of copyright infringement, forcing these farmers to pay fines and destroy their crop to prevent this spread.While this makes economic sense for these biotech companies due to the cost of research and development, this degree of ownership over something that naturally spreads is a big deal. This has the potential to make farmers significantly more indebted to these companies for their seeds, in a way that puts far more economic burden on small farms over large factory farms, and more burden on farms in the developing world than those in the developed world. And while there are reasonable safety concerns to GMO seeds and plants slipping away from human control and into the wild, it’s safe to assume that this was not Monsanto’s primary concern.
Another concern that comes up whenever genetic engineering is discussed is the concept of designer babies. Researchers have been working on using CRISPR to modify genes in human embryos for a few years now, altering the genes of a person before they’re born will be a possibility in the not-too-distant future. This has tremendous potential to fix genetic diseases and to reduce risks of other diseases. But it also has the potential to be used for cosmetic changes, making one’s future child smarter, stronger, or more attractive. If these kinds of cosmetic alterations are at all expensive, (which they will be at first) it could lead to wealthier children being innately “better” than poorer children, which would lead to better opportunities for these transgenic children which would lead to greater economic inequality. And of course, if genetic faults can be so easily fixed, there’s the concern of what counts as genetic fault. Neurodivergence such as Autism Spectrum Disorder is argued by many to be better thought of as a case of normal diversity in how human brains work instead of as a pathology. Historically, being LGBT+ was considered a disorder, and this is still an attitude with political sway in some parts of our society. If genes are discovered that influence these traits, what’s to stop parents with access to this technology deciding they don’t want their children to have them? What’s to stop them from deciding their children’s whole personality for them?
I’ve just scratched the surface of both of these topics. These two videos by Kurzgesagt (I honestly can’t recommend this channel enough) go into more detail about the ethical concerns about GMO crops and human gene modification than I have here and I encourage you to watch them both. But this should serve as a primer about how this technology could be abused.
My Thoughts
What follows is my completely subjective opinion on the ethics of genetic engineering, so feel free to take this with a grain of salt. That said, I have studied scientific ethics as part of my education as well as history of science and moral philosophy for my own interest, so...maybe not too much salt.
I tend to believe that new technology rarely if ever creates new problems. More often, it makes existing problems bigger. Before the invention of nuclear weapons, wars still killed and displaced civilians, destroyed infrastructure, and often did damage to the surrounding environments. Nuclear weapons didn’t create these problems, but they did make these problems substantially worse, to the point they could no longer be written off as ‘just the cost of war.’ And while it would be vastly incorrect to say that the advent of these weapons is the only reason why the world hasn’t seen a total war between major powers since their advent, it is reasonable to say that this was a contributing factor. Nuclear weapons made waging total war so terrible it became unsustainable, and at the same time, political infrastructure was created and how society thought about wars was collectively changed to reduce the risk of these conflicts happening again.
The general issues that I’ve brought up in this post include issues of the propagation of wealth inequality, abuse of intellectual property, the definition of disability, and bodily autonomy. These are all problems that our society is contending with today. Easy genetic engineering has the potential to make these barriers stronger and to create more opportunities for these abuses, but these are still old problems. And even if we ignore the more open-ended connections between these concerns and contemporary issues, there are a few direct parallels. As I mentioned before, genetic engineering isn’t too different from selective breeding, and this extends to legality. Plant breeder’s rights has been an issue of debate within copyright law for decades. Those who breed new strains of crops via selective breeding have long pushed against the use of saved seeds from their strains as it violates their copyright, but enforcing copyright this strictly would hurt farmers, particularly in developing countries. For the most part, GMO crops have been rolled into existing debates on plants as intellectual property, farmer’s rights, and seed sovereignty. Likewise, while parents can’t currently alter their newborn’s DNA, things like intersex surgery or cochlear implants mean that parents can make decisions for their children that fundamentally alter their future lives and identities. Hell, if you really want to fall down a philosophical rabbit hole, just having a child is to make a significant, life-altering choice for someone without their consent.
The optimist in me likes to believe that making these problems bigger or scarier could be a step toward finding solutions for them. If society can no longer ignore these problems, then it becomes easier to create policies and institutions to mitigate them. This...is probably just idealism; historically, this is rarely how it goes and there are usually other circumstances at play. As I said before, nuclear weapons are far from the only reason we haven’t had a world war in almost eighty years. But I do think that new technologies like this can create the opportunities for us to look more closely at the societal problems that these technologies will abut. This kind of society-wide reevaluation is by no means an inevitability, but if we use these opportunities to examine our own society and play our cards exactly right, technologies like this can have an overwhelmingly positive effect.
Ultimately, it will be a while before CRISPR-Cas9 can be used en masse by the general public. There are still concerns about off-target edits, where if you leave Cas9 proteins in a cell for too long, they’ll start to make random edits by chance. And it has always been much harder to add new genes to an organism than it is to take old genes out. Removing bad genes just takes a Cas9 protein targeted at said gene, the protein makes a cut, and the cell repairs the cut in a way that breaks the gene. Adding new genes requires a copy of the new gene and a Cas9 protein targeted the intended spot for the new gene, and the new gene needs to be right next to the Cas9 protein when it's making the cut so the cell repair mechanisms can use the new gene to fix the hole. But at this point, these are basically just engineering problems, and there are proposed solutions for both of them that just need to be proven effective in experiments. CRISPR-Cas9 will change the world, likely for the better overall, but possibly for the worse in a few notable ways. Let’s educate ourselves and support policies that help to mitigate this.
For More Details
https://urology.wisc.edu/wp-content/uploads/2020/10/Bacteriophages-Eur-Urol-2020-Leitner-et-al.pdf (Image 1)
Comments