Solar Storms
- WSP Rhodes
- Nov 19, 2023
- 11 min read
I recently read an article in the Washington Post about solar storms and how they are studied, and it reignited my curiosity for the topic. Solar flares and storms are a common doomsday scenario in fiction and thus are a topic most people have a peripheral awareness of even if they don’t fully understand them. So since peripheral awareness without understanding is my bread and butter at this point, and because there’s a solar storm occurring while I’m writing this (I didn’t plan that, I swear), let’s talk about solar storms, how they form, what risks they pose to human civilization, and if there is anything we can do to mitigate these disasters.
The Sun
I’m sure you’re all aware that the Sun is really hot. Having a mass over 300,000 times that of Earth, the Sun’s gravity crushes the material in its core with pressure 260 billion times that of Earth’s atmosphere at sea level. This high pressure, and the high heat that comes with it, triggers fusion reactions which heat the sun even further. The Sun’s core is over 15 million°C while the surface is a much cooler 7,000°C. At these temperatures, the hydrogen and other gasses of the sun turn into plasma. Plasma is the fourth state of matter, created when a substance becomes so hot that an atom’s electrons become too energetic to be held in place by the nucleus’ electromagnetic pull. Remember, atoms consist of a positively-charged nucleus ‘orbited’ by multiple negatively-charged electrons, and these charges cancel out to make the atom neutral. Plasmas are a bunch of charged particles that are too hot and energetic to coalesce and neutralize each other, like a bunch of magnets that are jumping around too much to stick to one another. And when charged particles move, they produce magnetic fields. Individual atoms have extremely tiny magnetic fields from their electrons whizzing around them that are normally too weak to detect, but when these tiny magnetic fields are perfectly aligned with each other, they reinforce each other to create permanent magnets. The Earth’s magnetic field is created by superhot-and-magnetic liquid iron flowing around inside the planet, pushed by convection and coriolis forces. These same forces push the charged plasma inside the Sun to create a magnetic field stronger than that of Earth. But on top of being stronger, the Sun’s magnetic field is much more chaotic.
When picturing a magnetic field, it’s useful to imagine a series of lines running from the magnet’s north pole to its south pole. It’s important to remember that these lines don’t start/stop at the magnet’s poles but instead pass through the magnet’s interior to create continuous loops. (See image below). Earth’s magnetic field works like this as well; a series of currents that enter the planet roughly around its south pole, pass through the core to exit at the north pole, then continue through the space around the planet in a loop to re-enter at the south pole.* Anything affected by magnetic fields, from charged particles in space to the needle of a compass, will follow these paths until they run into something that stops them.** The Sun’s magnetic field works like this too with one notable exception.

The Sun isn’t one solid object like the Earth, it’s made of a fluid-like plasma, which means it’s possible for parts of it to rotate at different speeds than other parts. The Sun’s poles complete one rotation every 34 days, but its equator completes one rotation every 25 days. Because the materials generating the Sun’s magnetic field aren’t moving in sync with each other, the magnetic currents inside the Sun get twisted like a coiled spring by this uneven rotation. When the Sun’s magnetic field gets more and more coiled and stressed, it violently realigns itself, causing storms in the process, before the Sun’s uneven spin starts to twist it again. This cycle of most to least twisted lasts eleven years with its exact mechanics and effects still being studied, but the leading theory of how exactly it works is as follows. At the beginning of this cycle, the Sun’s magnetic field looks much like the Earth’s, with a magnetic north and south pole and magnetic currents running straight between them. But over time, the uneven spin of the Sun twists these internal magnetic currents into a spiral. Like a spring being twisted too far, the Sun’s magnetic field becomes so stressed that it kinks, with parts of these kinks breaking the surface. Loops of magnetic force poke out of the Sun’s surface, creating a pair of smaller magnetic poles at the Sun’s middle latitudes with currents of magnetic force bridging these two poles. More and more kinks poke out of the Sun’s surface until the Sun’s original north and south pole becomes overshadowed and weakened. Instead of looking like a bar magnet with defined north and south poles on either end, the Sun is a tangled mess of magnetic currents connecting random points on its surface. But over time, these smaller poles are carried by thermal currents in the stellar plasma toward the Sun’s geographic poles. As these mini-poles collect around the geographic poles, they coalesce and form new magnetic poles. The Sun once again has an Earth-like magnetic field with defined north and south magnetic poles, though they’ve now flipped with the north pole being where the south pole was and vice versa.*** You might already know these mini-poles by their official name; sunspots. These magnetic kinks repel plasma away from the two points where they enter and exit the Sun, so these mini-poles don’t receive as much heat from the Sun’s currents. They cool down to below 3,000°C, which would still make them hot and bright enough to outshine the full moon if placed in the night sky, but on the surface of the Sun appear far darker. Scientists have observed sunspots for centuries and have found they follow a 11-year cycle, with fewer sunspots when the Sun’s magnetic field is orderly and more when it’s chaotic.

The period within this 11-year cycle where the Sun’s magnetic field is at its most tangled and sunspots are at their most prevalent is known as the solar maximum. For about a year, the prevalence of solar flares and storms goes from roughly one a week to multiple events a day. Solar flares are massive explosions on the Sun’s surface billions of times larger than human-made nuclear weapons which give off enormous bursts of x-rays and gamma rays. They occur whenever the tangled web of magnetic fields on the Sun’s surface suddenly snap back into place, like a really twisted rubber band returning to normal when released. There are several phenomena that can trigger solar flares, but the biggest are coronal mass ejections. CMEs begin from kinks in the magnetic field that break the Sun’s surface. These kinks can become full of stellar plasma, creating arches or loops of plasma on the Sun’s surface connecting two sunspots, so large the Earth could fit through them. These loops can be twisted even further by thermal and magnetic currents within the Sun, putting strain on the structure. There are multiple complex ways this structure can fail and the exact dynamics are still being studied, but one way or another, the loop becomes so twisted and kinked that part of it breaks off in order to restabilize the structure. Imagine you tried to tie a slinky into a knot, so it fixed itself by cutting the knotted bit out and rejoining the ends to create a shorter but more stable slinky. Unlike a slinky however, that cut-out bit contains billions of tons of charged particles and the immense energy created by the strain put on the original loop. Now cut off from the Sun and its magnetic field, this mass of plasma explodes outward into the solar system.
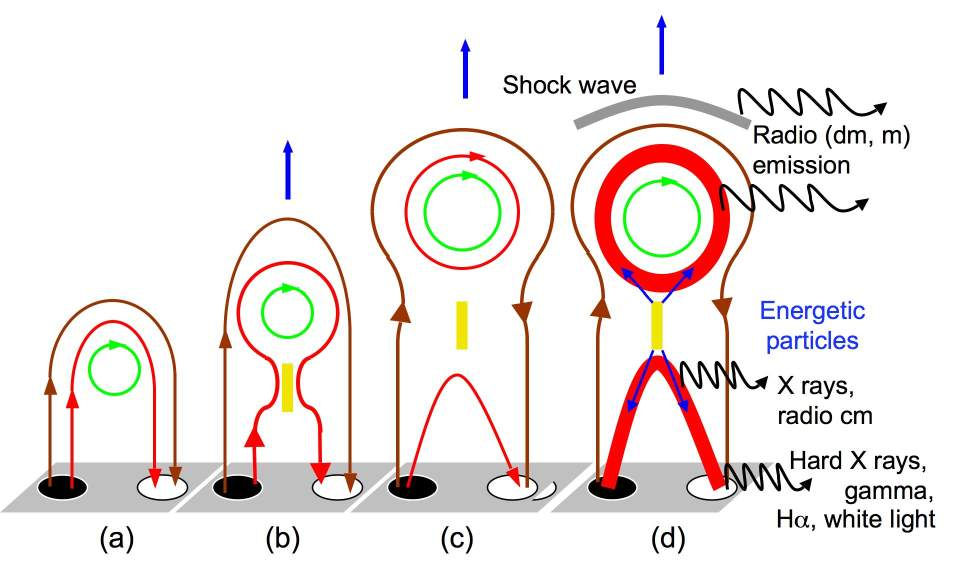
Diagram of part of a coronal mass ejection forming. A filament of plasma forms between two sunspots, part of that filament gets “pinched off,” and that removed part explodes outward.
Effects on Earth
The Sun is constantly ejecting charged particles into the Solar System, which we call solar wind. These particles are deadly radiation, posing health risks to astronauts and electronics without protection. Earth itself is protected from solar wind by its magnetic field and by its atmosphere. Earth’s magnetic field diverts the vast majority of charged particles away from the planet, with the majority of particles that do hit being channeled toward the north and south magnetic pole. These charged particles that reach Earth are stopped by its atmosphere way before they reach the ground. This is the origin of the aurora borealis, solar wind hitting the upper atmosphere and releasing their energy as light. These impacts with the atmosphere do knock atoms of air away from the planet, so over time solar wind could erode away a planet’s atmosphere (this is believed to be what happened to Mars, which doesn’t have a magnetic field and now has an atmosphere less than 1% as dense as Earth’s). But planets with magnetic fields deflect the vast majority of solar wind that hits them, so this atmospheric erosion is negligible. Earth will be destroyed by the Sun’s death long before solar wind erosion becomes a concern.
Solar wind does get stronger during solar maximums, but not by enough to be a significant concern on its own. Solar flares also add to the ‘weather’ of our solar system, but aren’t a significant threat. The sudden bursts of x/gamma rays created by solar flares can pose health risks to astronauts outside Earth’s atmosphere, and space agencies do monitor solar flares so they can calculate what doses astronauts are receiving, but these aren’t lethal doses on their own. Solar flares can also cause temporary disruption to short-wave radio transmission as they increase the ionization of the atmosphere which can absorb and refract radio waves. Outages like this are useful information to have so agencies like NASA monitor solar flare activity, but solar flares are not the scariest thing the Sun can throw at us.
When scientists talk about solar storms, they more often than not are referring to coronal mass ejections. CMEs release over a trillion kilograms of charged particles traveling at 3,000 kilometers per second. Should this tsunami of solar wind strike the Earth in just the right way, it can push against Earth’s magnetic field so much that it gets temporarily blown into a different shape. Moving magnetic fields induce electrical current, so a sudden shifting of Earth’s normally constant magnetic field induces electrical currents in the Earth’s crust, which in turn produces magnetic fields which induce currents in wires and circuits. These currents can be far stronger than what electrical equipment was built to handle, leading to short circuits and overheating which permanently damage or destroy equipment. The most vulnerable infrastructure to these induced currents is power grids, (since they already have current running through them and they’re continental size creates enormous induced current) but pipelines, phone lines, undersea cables, and railroads can also be affected. Additionally, CMEs can damage satellites by directly inducing lethal electrical current in them, since they’re outside the protection of Earth’s atmosphere, as well as temporarily disrupt radio communication and irradiate astronauts just like a solar flare. Personal electronics such as your phone or laptop would not be directly affected, they are far too small to produce the induced current needed to cause damage, but the loss of electricity and internet would indirectly render them useless.
Large solar storms have occurred in the past. The one you might be most familiar with was in 1989 as it caused a nine-hour blackout in Quebec. Quebec was particularly vulnerable to this storm due to a number of factors (high latitude, sitting on less conductive rock, long transmission lines to move this electricity from hydroelectric dams to population centers), but the storm hit the entire planet and caused power grid interference and radio blackouts around the world. The largest solar storm to occur since records began was the Carrington Event, three times as big as the Quebec storm which caused auroras as far south as Colombia and caused significant damage to electrical infrastructure all over the world. Fortunately, the Carrington Event occurred in 1859, so the damaged infrastructure was just nascent telegraph lines. But these failing telegraphs threw sparks, gave operators severe shocks, ignited fires in switchboards, and in one instance electrified the system so much that operators could continue sending messages even after switching off power to the lines. Given how much electrical infrastructure we have today, a storm the same size as Carrington, if nothing was done to protect against it, could cause approximately $2.6 trillion of damage in the United States alone (>10% of national GDP) and could take anywhere from several days to several years to bring power back online, depending on severity. During this time, multiple regions if not the entire planet could be without power, long-distance communication, and navigation, so they’d be without most economic activity and production, municipal water and sewage, or transportation needed to move food from farms to people. And Carrington isn’t even the largest solar storm we know to have ever occurred; ancient solar storms leave radioactive isotopes inside tree rings that act as a record for scientists (more on that here). The largest such storm we’ve found evidence for occurred 14,300 years ago and was at least ten times larger than the Carrington storm. If such a storm occurred today, it could be apocalyptic.
Prevention
So, how likely are we to experience an apocalyptic solar storm that destroys our civilization and knocks us back to the stone age? Less likely than you would think. How often these massive storms occur is still being researched, but Carrington-sized storms are predicted to only strike Earth once per century at the most frequent. Larger storms are even less common, potentially once per millennium if that. The majority of these storms are far weaker, with the storm last week being only one-fortieth the intensity of the Quebec storm. Such storms could create electrical fluctuations within power lines that have to be watched for and managed, but electrical grids already have to be constantly monitored for safety and stability reasons, so controlling for these fluctuations falls within normal practice. Also, since the 1989 storm that caused the Quebec blackout, reinforcing our civilization against these storms has become a priority for scientists and engineers. National weather services, such as the NOAA in the United States, monitor space weather using satellites and observatories to give advanced warning of radio blackouts and radiation hazards created by solar storms. CMEs can take hours or days to reach Earth, but they often create bright solar flares which reach Earth in minutes. We can usually see solar storms coming well in advance and respond by powering down satellites or reducing power plant production. Work has also been done to reinforce the power grid’s ability to survive these storms, such as shielding at-risk components, building systems that could absorb the energy from surges, frequently inspecting transformers and power lines to ensure they remain fit enough to survive a storm, and stockpiling transformers and other susceptible components to quickly replace damaged ones. Research is ongoing into better ways to predict storms and the exact ways damage is done to infrastructure to better understand the best ways to respond. But all the research so far is paying off; in late October of 2003, a series of solar storms hit Earth whose peak intensity was 80% as strong as the storm that caused the Quebec blackout. The extent of the damage this time was a one-hour blackout in Sweden and twelve transformers being taken out of service in South Africa. While not nothing, this is proof we are getting better at dealing with these storms. We have yet to experience a Carrington event-sized storm since the space age began so that will be the real test of our storm-proofing efforts. But we are far from helpless in the face of the Sun and of all the things we’ve talked about here that you should be worried about, this one is low on the list.
For More Details
*Counterintuitively, the Earth’s geographic north pole is actually closest to its magnetic south pole. The names of the magnetic poles come from how compasses work, with the north pole of the compass pointing northward. But north is attracted to south, so this means that the north of the compass is attracted to the planet’s south pole, which is near the planet’s north. Such things happen when you name things before you understand them.
**It should be noted that what direction these charged objects would move would be different for different charges. Positively charged particles would move toward the north pole while negatively charged particles would move toward the south pole along the same currents. Magnetic objects like metal or compass needles will align themselves with the magnetic field before being pulled toward the nearest pole, provided the field is strong enough and the object is light enough.
*** In case you’re interested, this flip happens because of where these mini-poles form. When these kinks break the Sun’s surface, the south mini-pole tends to form closer to the north pole than its partner does, and vice versa, due to the orientation of these magnetic field lines. This means the thermal currents will tend to deliver south mini-poles to the north pole, and vice versa. With the original poles weakened, these mini-poles overwhelm them and combine to form new poles with the opposite polarity.
Comments