Since this is my first post since I started working, I’m going to go easy on myself and cover a topic I am familiar with; genetics and disease. You’ve probably heard of at least a few genetic diseases, such as cystic fibrosis, Huntington’s disease, sickle cell anemia, muscular dystrophy, Ehlers-Danlos syndrome, or hemophilia. Genetics also plays a role in several other diseases, such as various forms of heart disease, high blood pressure, Alzheimer’s disease, autoimmune diseases, and certain cancers, which is why these diseases might ‘run in the family.’ I’ve written in past posts about how the genome works, but there’s plenty to learn from how it doesn’t work.
Single Gene Disorders
When you think of genetic diseases, this is almost certainly what you’re picturing; diseases caused by a single faulty gene. While I’m sure you all remember grade school genetics, let’s give a brief refresher on how genetic inheritance works. Humans have roughly 100,000 genes in their cells, each one being the instructions for how a specific protein is made. Each gene is located on one of 23 pairs of chromosomes, with each gene always being in the same location. Genes can have variants between different people which result in slightly different proteins, ranging in effect from causing a different hair color to breaking your immune system. All humans have two copies of each chromosome, one inherited from each parent, which means two copies of each gene.
If you remember grade school, you’ve almost certainly heard the terms ‘dominant’ and ‘recessive’ genes. Since you have two copies of all of your genes, it’s very common for these copies to be different and produce different proteins. In some instances, one of these traits will override the other trait for one reason or another. For example, lighter pigments of hair and eye color tend to be recessive traits because they can be easily overridden by darker pigments. If one were to have one gene for black hair and one gene for blonde hair, their hair would produce both pigments but only the black pigments would be visible. All of this was first cataloged by Czech polymath Gregor Mendel in his experiments with pea plants. He bred pea plants in his abbey’s garden as part of his job as an abbot, where he found that certain traits would disappear and reappear between generations. If a plant with purple flowers was bred with a plant with white flowers, all of the offspring would have purple flowers. But if these offspring were bred with each other, one-quarter of those offspring would have white flowers again. After experimenting with more than 28,000 plants over the course of decades, he’d identified several of these dominant-recessive trait pairings and developed a mathematical model to predict what traits would appear depending on the traits and ancestry of the parent plants. This research was the foundation of modern genetics.
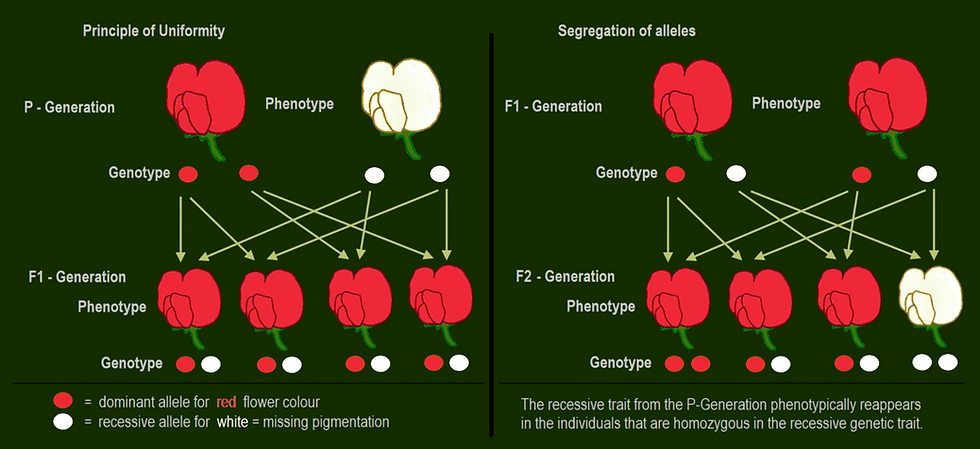
Understanding Mendelian inheritance helps with understanding genetic disorders as a lot of these single gene disorders follow Mendel’s models. The majority of single gene disorders are recessive traits, meaning one needs two copies of the disease-causing gene to get sick. These tend to be diseases caused by faulty single proteins, proteins where having half of them be defective isn’t a problem because the other half work fine. A good example would be cystic fibrosis, caused by having two broken copies of the gene CFTR. CFTR normally sits on the surface of a cell and regulates how much salt enters and leaves. Without functioning CFTR, cells accumulate too much salt and start retaining water, leaving less water available for one’s bodily fluids. The lungs normally have a thin lining of mucus to trap dust and irritants, but the mucus in cystic fibrosis patients is abnormally thick and sticky, which obstructs breathing, causes inflammation, and serves as a breeding ground for bacterial infection. Recessive diseases are the ones where parents can be a carrier, meaning they have one bad copy of the relevant gene but one good copy, so they don’t experience any symptoms. If two parents who are carriers have a child, there’s a one-in-four chance of the child inheriting both bad copies and having the disease.
Genetic disorders caused by dominant traits are less common, but they still exist. These are the diseases caused by structural proteins, proteins which attach to each other like puzzle pieces to form larger structures. Having one bad copy of one of these genes is like having a house where half of the bricks are made of styrofoam; the fact that half of them are normal bricks won’t give you a half-structurally sound house. A good example of this is Marfan syndrome, caused by having at least one broken copy of the gene FBN-1. This gene produces fibrillin, an elastic protein that is a component of multiple connective tissues. Patients with Marfan syndrome will have connective tissue which is too flexible, which leads to limbs growing abnormally long, joints being easier to dislocate, scoliosis, the lenses of the eyes being easily dislocated, and structural problems with the heart, lungs, and nervous system that can impact their functionality. Dominant diseases don’t have carriers; anyone with the disease-causing gene will have the disease. Those with only one broken copy of the gene have a one-in-two chance of passing it to their children while those with two broken copies can only produce children with the disease.
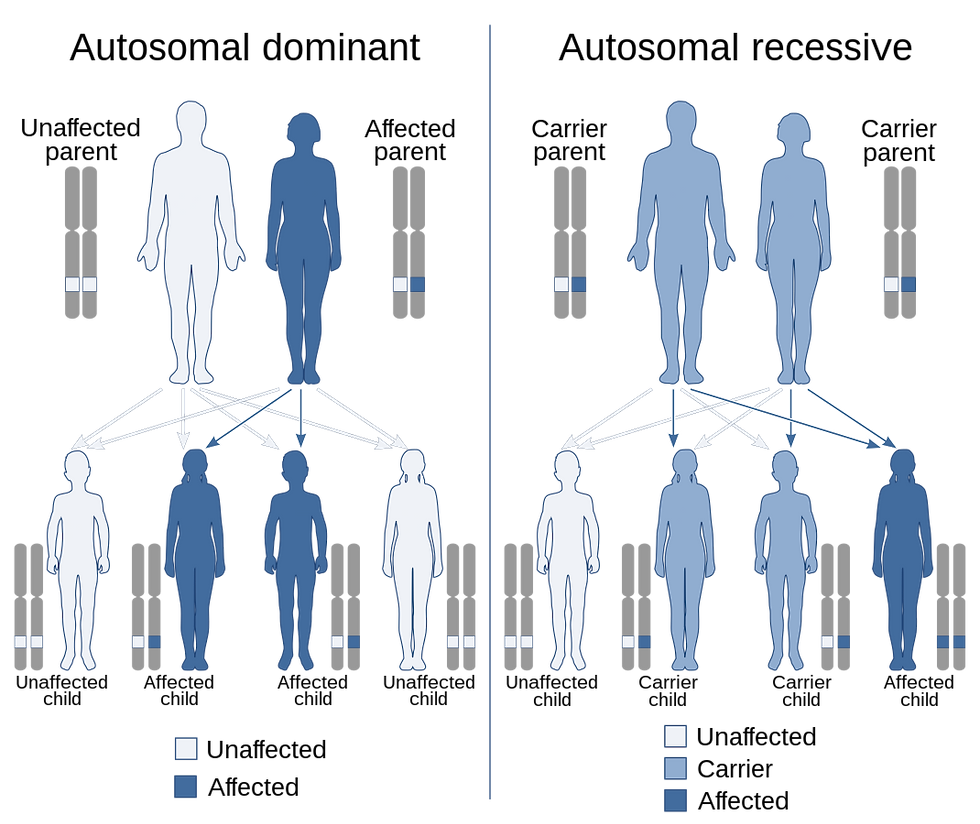
It should be noted that Mendel’s model of dominant and recessive traits don’t describe all genes, in fact only a minority of genes follow this system. Traits that follow this logic were the easiest to study for Mendel, but the majority of traits are codominant or show incomplete dominance. For these traits, having two different genes results in having both traits or a trait that’s halfway between the two traits. This would be akin to a flower with one red gene and one white gene producing pink flowers or flowers with red-and-white splotches. Many diseases follow incomplete dominance, with a patient having one disease-causing gene results in mild symptoms while having two results in more severe symptoms. There are several diseases like this, with some being considered dominant or recessive depending on how severe the one-gene version of the disease is. Familial hypercholesterolemia (causes dangerously high cholesterol due to the liver’s inability to clear it) and sickle cell anemia (misshapen red blood cells resulting in multiple symptoms) are both technically incomplete dominance diseases, as they both result in milder symptoms with one gene and worse symptoms with two genes. But familial hypercholesterolemia is generally considered to be caused by dominant inheritance because the one-gene version can still result in an abnormally high risk of heart disease, while sickle cell anemia is considered a recessive disease because having one copy of the gene only results in mildly misshapen blood cells that rarely cause symptoms outside of scuba diving. And I won’t go into the other ways single gene disorders can become complicated, such as X-linked inheritance or chromosomal abnormalities. Just know that even in its simplest form, genetics is complex.
Multifactorial Diseases
While single gene disorders are probably what you think about as a genetic disorder, they actually represent a minority of genetic diseases. This makes sense when you think about it; if one mutated gene was all it took to have a debilitating condition, that gene would be weeded out of the genepool pretty quickly. This is also why most single gene disorders are recessive traits; most people with the disease-causing mutation will be carriers instead of patients, and carriers can reproduce just as well as non-carriers. In fact, very often the mutations causing single gene disorders, especially dominant traits, are new mutations. When a genetic mutation occurs in an egg or sperm cell, that mutation will become a part of the genome of the person it creates. And there are certain genes that are ‘easily misspelled’ so to speak. Of the 10,000 people with Marfan syndrome, 25% are the result of a new mutation; neither parent had Marfan syndrome, but a sperm or egg happened to have a broken copy of FBN-1. Of hemophilia cases, 30-33% are caused by spontaneous mutations, which happened to Queen Victoria and caused hemophilia in many of her descendants. (If you’re interested, this video by Lindsay Holiday talks about this history and a little bit about genetics that I did not go into here) When these spontaneous mutations occur, they have a higher chance of being removed from the genepool by natural selection, so the inherited variants that cause single gene disorders tend to be rarer and relatively recent. For a disease-causing variant to stick around for a long time and become common, it needs to be subtler.
Multifactorial diseases are those that are caused by multiple genes, none of which alone will cause severe symptoms but together can. Diseases like Alzheimer’s, schizophrenia, epilepsy, diabetes, multiple birth defects, and many, many more fall under this category. These diseases won’t have any one gene tied to them, but they can run in families or be more common among certain ethnic groups, all signs of a genetic cause. For Alzheimer’s disease, scientists have identified over 70 associated genes, with certain genes having variants which could slightly increase a person’s risk of developing Alzheimer’s while some genes have variants which could all but guarantee developing the disease. Keep in mind that ten years ago, the number of discovered genes was only ten, so it's very possible we haven’t found them all yet. Schizophrenia has 287 genes associated with it while 222 genes have been identified so far associated with one’s risk for Type II Diabetes. None of these genes definitively cause these diseases, but they can increase one’s risk of developing these conditions.
Multifactorial diseases tend to be continuous traits, meaning everyone falls somewhere on a continuum of how susceptible they are. Unlike single gene disorders or infectious diseases where one either has the disease or doesn’t, multifactorial diseases can have a lot of gray area. The best way to think about them is in terms of points; having certain variants of certain genes give or take away a certain number of points. For diseases like Alzheimer’s, there is a certain number of points a person can have where symptoms will begin to manifest, and the more points they have past that threshold, the worse those symptoms will be and the earlier in life they will manifest. Alzheimer’s is an example with a very distinct threshold, but for others it can be even grayer. Obesity, heart disease, hypothyroidism, and depression are all diseases of gradation, where symptoms can be so light they barely count as a disease and there isn’t a distinct boundary between someone having the disease and not. And then we get into diseases where points basically just mean probability; certain genes make you more likely to develop cancers, autoimmune diseases, and allergies. And all these definitions blend together to create a complex scale of points, where a few points translate to a low probability of getting ignorable symptoms while a lot of points translate to a high probability of debilitating symptoms. And this isn’t even touching on how environment and lifestyle can also add or remove points. Since no one gene is responsible for someone developing a disease, they can remain in the genepool for a long time.
This lack of definitive genetic responsibility makes studying these diseases difficult. I’ve talked before about how the majority of proteins are just cogs in incredibly complicated machines, which makes understanding what each cog contributes to the machine incredibly difficult. What has allowed scientists to research multifactorial diseases has been mass data collection and processing. The genomes of patients are collected and compared against thousands of others. With so many data points, computers can identify gene variants that appear more often in disease patients than those that are healthy. Very often scientists won’t understand how a protein contributes to a disease or how it is supposed to work normally, but they can begin to infer its function from what happens when it breaks. This has led to new avenues of symptom management for those with these bad genes. Patients with a family history of certain illnesses or cancers can now have their DNA tested to determine their personal risk. From there, lifestyle choices such as diet and regular medical screening can be used to remove points. While this can be beneficial for illnesses like heart disease or diabetes where treatments do exist, testing for untreatable diseases like Alzheimer’s presents a moral dilemma. Every ethics class I took in my graduate program raised the question of whether or not it's right to test for a disease that can’t be treated.
Fortunately, effective treatments for genetic disorders are being developed. Traditionally, treatment consists of symptom management and medications which replace proteins the body can’t produce themselves. These treatments have been a godsend for patients (look up what happened to diabetes patients before the discovery of insulin), but these are still lifelong conditions. With the development of gene therapy however, there might be ways to undo genetic disorders. The use of genetic engineering to treat disease has existed in some form since 1990, when four-year old Ashanthi DeSilva had her SCID (bubble boy disease) successfully treated using gene therapy. SCID prevents T-cells from forming properly resulting in severe immunodeficiency, so doctors isolated a few of DeSilva’s defective T-cells, genetically altered them to function properly, and reintroduced them to her body where they reproduced into a functioning immune system. This worked because T-cells can be isolated and reintroduced to the body unlike many other tissue types, but advances in gene delivery have been made since 1990. Currently, there are over 20 different gene therapies approved by the FDA, used to treat single gene disorders such as hemophilia and muscular dystrophy, as well as treating certain types of cancer. There are many methods used in these therapies, but the most common involves inserting the healthy gene into a virus which can deliver the gene to a target tissue where it can then be incorporated into the patient’s genome. With technologies like CRISPR, it could be possible to do this very accurately with few side effects. These therapies are still in development and are extremely expensive, carry certain risks, and are only available for single gene disorders. But this technology will only improve, so we may soon be able to treat what was once untreatable.
Comentarios