Climate Change, How Do We Know?
- WSP Rhodes
- Nov 15, 2020
- 10 min read
Well, this is a blog dedicated to science education, so it was inevitable that this topic would come up eventually. Today, we’re going to talk about climate change. I’m sure most of you are already familiar with the basic science of climate change; burning fossil fuels releases carbon dioxide, carbon dioxide traps heat in the Earth’s atmosphere, and enough fossil fuels have been burned over the past couple of centuries to significantly increase the Earth’s average temperature. But I’m going to focus on the methodology behind this science; how we know what we know, what this knowledge means, and what the consequences of this knowledge realistically are. My hope is that this will be the best way to dispel some of the myths and refute some of the bad faith arguments that have unfortunately stifled much of the conversation about this extremely serious problem. So without further ado:
How We Know What We Know
Temperature and Climate Data
The most obvious data that Earth’s climate is changing is the sundry meteorological data that has been collected over the past century. Networks of radio-connected weather stations have existed since the 1930s. The mass standardized collection of weather data has been going on since the 19th century, so we know what the weather was like a century ago. Since the late 19th century, global temperatures have risen roughly 1.14 degrees centigrade (we’ll talk later about what this means) and are rising faster as time goes on. The oceans have warmed an average of 0.33 degrees centigrade (0.6 Fereinheit) and have risen about 20 centimeters (8 inches). Ice sheets and glaciers have been shrinking every year for decades while sea ice has been declining as well. Extreme weather events have been increasing in number and severity, such as hurricanes and droughts. Now in fairness, this evidence only proves that climate change is occurring, not its cause. For that, we should talk about…
Ice Cores
You might have seen this in a few films, where scientists in parkas drill into the ice with a cylindrical drill to extract a long pole of ice. These are ice cores; cross-sections of a glacier or ice sheet that show its history. Ice sheets (land ice found in Antarctica or Greenland) occur wherever more snow falls than melts, which means snow builds up over time. As it builds up, the snow on top crushes the snow on the bottom, turning individual flakes into a single sheet of ice. But snowflakes are light and fluffy, which means there is air between the flakes. As they get crushed, there’s nowhere for this air to go. So these spaces between the flakes get crushed down into microscopic bubbles of air, frozen in the ice. These bubbles contain atmosphere from when that snow first fell and because this process is so slow, these bubbles can be truly ancient. The Antarctic ice sheet is 4,000 meters at its thickest (13,000 feet or 1,300 stories) meaning there’s air trapped at the very bottom from as far back as 34 million years ago (though the oldest samples that have actually been extracted are only 2 million years old. Turns out it’s hard to drill out a core ten times the height of the tallest building in the world.). The air trapped in these bubbles can then be extracted and analyzed, showing the atmospheric composition when that snow first fell. Using this method, climatologists can track the fluctuations in atmospheric carbon dioxide over the course of hundreds of thousands of years. They’ve found that carbon dioxide in Earth’s atmosphere has increased from 280 ppm before the industrial revolution to 412 ppm today, a significant change over a very short period of time.
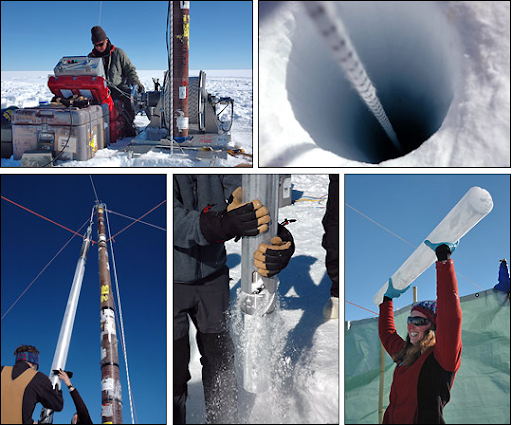
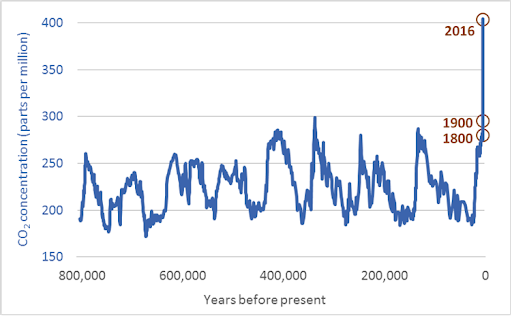
Carbon-13 Ratio
Isotopes and radioactivity would be a good topic for another time, but the shortest possible version is thus; the nucleus of all atoms are made up of protons and neutrons. The number of protons in an atom determines what element it is; any atom that has six protons, no matter what, will have all the chemical properties of carbon. On the other hand, the number of neutrons doesn’t affect the chemical properties, so you can find atoms with different numbers of neutrons. That said, there are isotopes that are more stable and common than others; the most common isotope of carbon is Carbon-12 (6 protons, 6 neutrons) at about 99% of all carbon atoms, followed by Carbon-13 (6 protons, 7 neutrons) at about 1% of all carbon atoms. The only real difference between the properties of these two isotopes is that atoms of Carbon-13 are slightly heavier than atoms of Carbon-12. Because of this slight difference in mass, living cells prefer to use Carbon-12 as a building block as it is easier to move around and use. This results in a different ratio of isotopes between organic and inorganic carbon. Carbon that doesn’t come from a living source, such as diamonds, graphite, or carbon dioxide from volcanic eruptions, has a ratio of roughly 93 Carbon-12 atoms for every 1 Carbon-13 atom. But carbon that comes from living sources, including fossil fuels and carbon dioxide released from organisms, will have even less Carbon-13 (between 1% and 3.3% less Carbon-13 depending on what species it came from). This means that you can look at the ratio of Carbon-12 to 13 and know more about its source. In short, the less Carbon-13 relative to Carbon-12, the more the source of CO2 is from organic sources.
Earth’s atmosphere today has proportionally less Carbon-13 than it has had in the past. Ice core samples from before the industrial revolution show that all the CO2 added since then has come from organic sources. This means that this extra carbon dioxide couldn’t have come from volcanic eruptions or any other geologic activity. Coal and oil both count as organic sources of carbon; they are the fossilized remains of 300 million year old trees and algae, so their carbon follows the isotope ratio of living carbon. From this, we know that the extra CO2 in Earth’s atmosphere, that we know is warming the planet, comes from the burning of organic material, and we’ve been burning massive amounts of 2°C organic material for over a century.
Average Temperature
You may have heard climatologists use numbers like 2°C of temperature rise and been confused as to why only two degrees is a big deal. The important thing to remember is that this is the global surface temperature change. Since the beginning of the industrial revolution, the Earth’s average temperature has risen 1°C (1.8°F). This means that if one takes temperature measurements all across the land and the surface of the ocean and averages them all together, that average would be about one degree warmer than it would’ve been two centuries ago. Please note that variance is important whenever one is talking about averages (the average human being after all has one ovary and one testis). So a rise of just 2°C above pre-industrial levels would still be a big problem. While it is extremely difficult to predict exactly what temperatures will be in different parts of the world, 2°C means that summers in the tropics could be tens of degrees warmer on average with heat waves even higher than that. But because other times and places might have less than one degree of warming and some might even become slightly cooler, the global average is only two degrees warmer.
Figuring out exactly what temperatures and precipitation will be in a warmer future is astronomically difficult. We can barely predict the weather a week from now, much less the weather a century from now. But just because we don’t know specifics doesn’t mean we can’t deduce trends. For instance, I can’t tell you what the temperature will be on New Year’s Day of this year; at time of writing, it’s about two months away. But based on seasonal cycles, I can tell you it will likely be colder than it is right now where I live. With other data, such as historical temperature data and possibly some data about atmosphere thermodynamics, I might be able to guess the temperature within about ten degrees of accuracy. Just like with Covid, experts are working with incomplete data on a complex problem at the very frontier of human knowledge. But just because they don’t know everything doesn’t mean they know nothing. Even if we don’t know what the weather will be in a century, experts understand the trends well enough to know it will be very different from today’s.
What This All Means
A common bad-faith criticism of climate models is, “the climate has changed before, how bad can it really be?” First off, while the climate does periodically shift and oscillate, it usually doesn’t do so this quickly. This comic by Randall Monroe’s xkcd is a great illustration of how the change we are currently going through is far faster than any the Earth has seen in the past 200,000 years (read all the way to the bottom). Slow climate changes, like that of the end of the recent ice age, can radically change an ecosystem as niches change and the species that filled these niches shrink in population, sometimes going extinct. Fast changes can lead to mass extinctions where numerous species go extinct because nothing can adapt fast enough to the changing climate.
It is true that the Earth itself can weather human-made climate change; it’s already survived much worse. It’s also true that the human species will likely weather this as well, as our ancestors managed to survive the end of the ice age. The question is how will our society and individual humans weather a rapid change that could occur in a lifetime (approximately 80 years). For example, all city infrastructure is built with a specific band of temperature and precipitation in mind. But as the climate shifts, existing infrastructure stops fitting with the new climate. The first example that might come to mind are anti-flood measures; sea walls, levees, and drainage systems that have to be built in places they weren’t before. But there are more subtle examples of how cities are built for their specific climate.
For example, almost all buildings in Europe are built to trap heat and without air conditioning. This has become a problem in recent years as summer heat waves have become more common, with one in 2019 killing over 800 people. Many cities, such as Vancouver, Los Angeles, and Las Vegas, get most of their water from snowmelt; snow falls on nearby mountains in the winter and melts over the course of the year. A concern in recent years is that as more winter precipitation falls as rain instead of snow, the reliability of this water source will be lost and new sources will have to be found. And the exact mixture of materials used to construct everything from roads to electrical lines to water pipes are determined with temperature in mind. An unplanned-for rise in temperature can make them more prone to wear-and-tear, requiring they be repaired more often or rebuilt with the new local climate in mind, both of which would be extremely expensive. These critical pieces of infrastructure and many more will have to be adapted if the climate significantly changes, which will be extremely expensive and might have to be redone if the climate continues to change.
But cities are not the only sources of infrastructure which are climate dependent. Where farms are located are extremely dependent on climate. Modern agriculture requires a lot of fresh water that isn’t too seasonal and can be controlled. As rain patterns shift due to climate change, most of our existing arable land will see reduced yields if not complete desertification. Now, new arable land will also be created in places like Canada and Russia, as land that’s currently too cold to be arable warms up. But this would require abandoning almost all of our existing farmland and rebuilding infrastructure in new places. This wouldn’t just mean the farms themselves, but also dams, aqueducts, and complex road networks to transport everything. Going back to cities (and residential regions in general) they too are dependent on steady access to fresh water. Desertification, along with rising sea levels and even more frequent heat waves, could cause some cities and regions to depopulate as they become less hospitable. This might mean that urban centers in these newly warmed-up regions will have to be greatly expanded and new ones might have to be built. Again, this is technically doable, but it would be very expensive. To bring this down to an individual scale, imagine having to sell your house in a region that everyone is trying to leave and buy one in a region that half the continent is trying to move to.
And of course, there is the issue of how such rapid change to the environment around us will affect the political stability of nations. The Pentagon listed climate change to be the biggest long-term threat to the United States’ national security. For example, there is evidence that the Arab Spring movement might have been in part triggered by years of record-breaking drought in the Middle East. This drought led to the collapse of local farms, leading to farmers migrating to urban centers, mass unemployment, and a widening wealth disparity which deepened anti-government sentiments. This is certainly not to say these movements were solely caused by climate change, merely that these factors could’ve been the straw which broke the camel’s back, leading to a movement whose effects are still being felt almost a decade later. Additionally, water rights have been a major source of contention in the Israel-Palestine conflict and Pakistan has also been hit hard by routine droughts. Do remember that Israel (allegedly) and Pakistan are nations with nuclear weapons, meaning any government instability or collapse has the potential to be catastrophic. If this all seems too far from home, consider this; the United States has one of the largest agriculture industries in the world. A significant warming of the climate would cause most if not all of America’s arable land to turn into desert while new farmland is created in parts of Canada. Losing this farmland would reduce America’s food security and cost about 10.9% of the nation’s jobs. So...how would the United States respond to this sudden loss of food security? How would this affect relations between the United States and Canada?
These are only a few examples of the way manmade climate change could impact our society in as short a time as a single lifespan if we remain on our current trajectory. The goal of the 2016 Paris Climate Accord was to keep warming from surpassing 1.5°C above pre-industrial levels by the end of the century, with 2°C being a secondary goal. To oversimplify, 1.5°C of warming would mean average temperature rising in most places but with little change to the areas’ biomes. Even 2°C of warming would mean deadlier heat waves and hurricanes, a significant reduction of crop yields in the tropics, and sea rise significant enough to completely flood some island nations. If nothing is done at all, we’re looking at 4 to 5°C of warming by 2100. To put this in a bit of perspective, during the coldest part of the last ice age, when the arctic ice sheets extended as far south as St. Louis, it was roughly 4.5°C colder than it is today. Continuing the warming under 1.5°C is a very optimistic goal; it will require significant international cooperation and major policy changes, possibly even reconsidering some core tenets of our economic doctrine (which might be why so many people in power would like for this to all be a hoax). But the point I hope I’ve made here is that the cost of continuing the warming under 1.5°C pales in comparison to the costs we’ll have to deal with if we can’t. This is a storm we probably can weather, but should we if we can avoid it?
For More Details
Comments