Cancer Basics
- WSP Rhodes
- May 9, 2021
- 10 min read
Forgive me for my brief hiatus, I have been busy with my classes and my graduate project.
This week, I thought I would branch out into other diseases and give a very high level description of the genetics and physiology of cancer. I’ve found that cancer is a subject that is less well understood by the public than its prevalence would suggest. For one, cancer can better be thought of as a group of related diseases instead of a single disease. There will likely never be a single ‘cure for cancer’ for the same reason there will likely never be a single ‘cure for viruses.’ To understand this variety, let’s start with…
Mutation
We’ve talked a lot about genetic mutation before, both in viruses and in multi-celled organisms. To reiterate, every time a cell divides, it has to copy its entire genome so both daughter cells can have identical copies. (See Diagram below) But the process of replicating DNA isn’t perfect, and sometimes spelling errors occur. We’ve only really talked about what happens when a mutation occurs across a whole organism, such as when a mutation occurs in a sperm or egg cell that grows up to be a person with the mutant gene in their every cell. What happens when a mutation occurs in one cell on a grown human body, making that cell different from every cell around it?
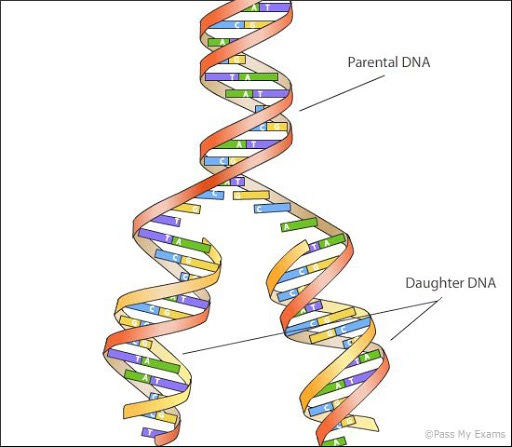
In the vast majority of cases, the answer is basically nothing happens. Cells have the ability to detect and fix mutations, so 99.9% are fixed as soon as they crop up. Even when a mutation is missed by this repair system, the majority of mutations won’t do anything or will only cause this one cell to not work properly. Very rarely however, a mutation will occur on either an oncogene or a tumor suppressor gene. A tumor suppressor gene is any gene that’s part of the cell’s DNA repair mechanism, so mutations here can break this mechanism and cause all mutations to go unfixed. An oncogene is any gene that is related to the rate of cell reproduction, so mutations here can cause the cell to reproduce faster, which creates more opportunities for new mutations with each new cell. In either case, mutations can dramatically increase the risk of further mutation.
In any multicellular organism, cell-to-cell cooperation is extremely important. There are numerous genes in the human genome that determine when cells grow, how they behave, and how they interact with each other. Part of the reason we all grow from single cells is so that every cell in our bodies is genetically identical, thus having identical copies of this genetic rulebook. By communicating with each other using certain hormones, cells can coordinate where and how often they reproduce in order to grow at a pre-programed speed and arrangement. But when any cell in the body becomes more prone to mutation, it becomes more likely that the genes that govern this ruleset will start to break. As a cell stops listening to these growth rules, it starts reproducing faster, with each daughter cell becoming a new opportunity for mutation.
Becoming Cancerous
Now, this process of going from one mutation in one cell to an uncontrolled tumor can take a long time. Part of the reason that cancer risk increases with age is that it can take a lifetime for these mutations to build up. Ultimately, cancer is a disease of probabilities; every cell has some odd of having the first mutation, which makes the second mutation more probable though potentially still unlikely. The reason cancer is so prevalent is because the human body is made up of roughly 40 billion cells, so the odds that any one of them becomes cancerous is still reasonably high.That said, there are multiple factors which can affect the probability of a cell mutating into a cancer. For example:
Tissues that reproduce faster than others (bone marrow, stomach and intestinal lining, immune cells) are more prone to developing cancers as their fast reproduction creates more opportunities for mutations.
Tissues associated with sex organs (breast and prostate tissue) are also more prone to cancers as they are already programmed to respond to certain hormones. Androgen and estrogen are growth hormones, so the tissues that respond to them don’t have to break the rules, just listen closer to specific rules, which is a more likely mutation to occur.
Being exposed to certain things can increase one’s cancer. Several forms of radiation and chemical carcinogens, such as those in cigarette smoke, asbestos, and numerous others can cause DNA damage which leads to mutation when this damage isn’t repaired properly. Those who work in industries or live in areas where they are regularly exposed to these substances can be at higher risk of certain cancers.
It’s also possible to inherit certain mutations that make certain cancers more probable. The gene BRCA1 is a part of the DNA repair system that you might have heard of due to its link to breast cancer. Most people have two copies of this gene in every cell, meaning both copies have to break simultaneously in the same cell in order to put someone at risk. But it’s possible to inherit one broken copy of BRCA1 from a parent, meaning each cell has only one functioning copy of the gene. Any cell only needs to lose that one copy to become precancerous.
No matter what, it will take many mutations for a tumor (a mass of mutant cells all descended from the original mutant cell) to become cancerous. Tumors are only considered cancerous when they’ve lost all control mechanisms on their reproduction and are growing uncontrollably. But even before this point, the cells of the tumor are still mutating as they rapidly reproduce without repairing mutations. All tumors are heterogeneous, meaning no two cells are exactly alike due to all the mutating. And like bacteria, if one tumor cell has an adaptation which makes it survive better than its neighbors, then that cell will have more descendants to spread that adaptation to. This allows the tumor to adapt to its surroundings and solve problems.
Firstly, the tumor needs to secure an oxygen supply. In normal tissue, the circulatory system grows with the tissue to ensure every cell is close enough to a blood vessel to receive enough oxygen. But when a tumor grows without instructions from the surrounding tissue, blood vessels don’t grow with it. These benign tumors can only get about one millimeter across before their centers start to die from hypoxia. This can be big enough to cause health problems if they’re in the right spot, such as the tightly packed cells of the brain. (Fun fact; the accepted reason for why people don’t grow new brain cells after childhood is because this makes brain tumors harder to develop.) But for the majority of tissues, tumors need to adapt to this obstacle before they pose a threat. Now, cells can secrete growth factors that cause new blood vessels to grow. These growth factors are normally highly regulated, but these tumor cells stopped caring about that long ago. Once one tumor cell mutates just right to start secreting these growth factors en masse, new blood vessels will grow into that part of the tumor. These new blood vessels are often called ‘leaky’ blood vessels; they’re built so quickly and haphazardly that they’ll have gaps in them and are missing some of the ‘infrastructure’ usually found in healthy blood vessels. Research is being done into how to treat cancers by targeting these very distinct leaky blood vessels, but this is still ongoing.
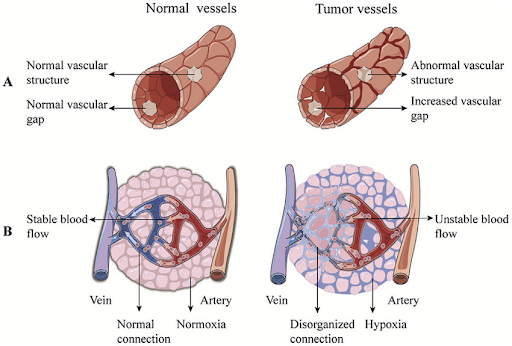
Once blood is flowing through the tumor, it becomes easier for the immune system to identify these tumor cells and attack. The majority of cancers that get this far will end this way as the immune system has cells which can identify and destroy cancer cells. But if there are any tumor cells with mutations that let it hide from the immune system or reproduce faster than it can keep up with, they can hide from the immune system indefinitely. With a few more mutations, the tumor can grow without restriction. This tumor can then damage the body by stealing nutrients and oxygen from other tissues, secreting toxins, putting mechanical stress on surrounding tissues, or stop organs from doing their jobs. With enough time, tumor cells can become dislodged from the original tumor, travel through the bloodstream, and create new tumors elsewhere in the body. This is called metastasis and makes treatment far harder.
Treatment
As I’ve said before, cancer isn’t a single disease; it’s an umbrella term for any disease caused by cells mutating and growing out of control. Even within one cancer ‘type,’ there can be a lot of variation because most organs aren’t made of one type of tissue. For example, breast cancer might mean a ductal carcinoma (the original mutant cell was from the milk duct) or a lobular carcinoma (the original cell was from the milk gland). You may have heard the terms ‘small cell lung cancer’ and ‘non-small cell lung cancer’; small cell refers to a particular type of fast spreading cancer of the bronchial tubes while non-small cell is an umbrella term for slower spreading, less scary lung cancers. Leukemia and lymphoma are both blood cancers, but lymphoma forms when cancerous blood cells get lodged in a lymph node and grows into a solid tumor while leukemia is a liquid tumor, where cancerous blood cells crowd out healthy blood cells, leaving the body without blood. On top of this, cancers can be different due to what their original mutations were. Depending on what the initial mutation was, new mutations can take the form of genes breaking, genes being switched on or off at the wrong time, or having the wrong number of certain genes due to weird replication. This can affect the shape of cancer cells, how fast they grow, and to what drugs they respond.
All of this can affect how these diseases are treated, from what drugs are used to how aggressive the treatment is. You’re probably familiar with the traditional methods of cancer treatment, such as surgery, radiation, and chemotherapy. Chemotherapy refers to a number of different drugs which are used to treat cancer because they are most effective against rapidly-reproducing cells. While there are many mechanisms by which to do this, these drugs usually cause temporary damage to a cell’s DNA, which can be fixed so long as it’s done before the cell replicates. Cells that replicate quickly make copies with the damaged DNA, causing them to die. This is very effective against cancer cells, but also affects the body’s other rapidly replicating tissues. This is why chemo has so many side effects such as hair loss, nausea (from the loss of stomach lining), and a weakened immune system (from loss of immune cells). Another disadvantage of chemo is that, like with antibiotics, tumors can develop resistance to drugs. If any tumor cells have a mutation which makes a particular drug less effective against it, it will survive the treatment and repopulate the body with its descendants. There are strategies to prevent this, from using several different chemo drugs at once to how dosing is scheduled, but nothing is perfect. If a cancer recurs (a few tumor cells survive the treatment and repopulate the body) the drugs used to treat it originally will almost certainly be ineffective.
Fun fact: the first chemical agent to be used for chemotherapy drugs was nitrogen mustard, which began being used to treat lymphoma in 1942. If that name and date sound familiar, it's because nitrogen mustard is chemically similar to sulfur mustard, the active ingredient in mustard gas. During World War I, it was observed that soldiers who were exposed to mustard gas and initially survived would sometimes die two to three months later. Autopsies showed that all their bone marrow had died, leading to a loss of red blood cells and immune system collapse. Bone marrow is the fastest growing healthy tissue in the body and is where blood cancers such as lymphoma originate. So after a few decades of research, drugs using nitrogen mustard were used to treat lymphoma. These drugs were incredibly toxic, especially by today’s standards, but untreated lymphoma can kill a patient in weeks, so even injecting a patient with mustard gas counted as an improved condition.
In hindsight, this fact was not particularly fun.
Fortunately, cancer treatment has improved significantly since 1942. In the past few decades, we’ve started to see the emergence of personalized medicine in cancer treatment. Personalized medicine is an umbrella term for treatments that are tailored to a patient’s unique body and needs. Using information about a patient’s genomics, transcriptomics, and several other types of omics, doctors can learn the exact mechanism of a patient’s illness, how an illness will affect them, and what drugs and doses will work best to combat this very specific case. Personalized medicine has a lot of applications in every field of medicine (I personally took a DNA test to be matched to the specific medication that I have been taking for several years now). And due to how varied and unique every cancer is, oncology has made great use of personalized medicine techniques. It’s not uncommon today for cancer patients to have their tumors genotyped in order to identify weaknesses to exploit. Such as;
I previously mentioned that certain breast cancers are caused by tumor cells becoming more responsive to estrogen than healthy cells. Drugs that block the body's production of estrogen can slow or stop these tumors' growth with very few (but still some) side effects than traditional chemo. The same is true of certain uterine and prostate cancers.
Immunotherapy for cancer might be something you’ve heard of. If tumor cells have proteins that aren’t found on healthy cells, it’s possible to harvest these proteins and use them to develop a personalized vaccine which can help the immune system to target cancer cells.
Targeted therapies are probably the closest to what one would think of as personalized medicine. As mentioned, there are many different ways that two different cancer patients can have different cancers, even if both are in the same tissue of the same organ. Depending on what genes mutated and in which order, there could be a way to stop tumor growth using relatively simple drugs. For example, up to 90% of (certain types of) leukemia cases start with a mutation called the Philadelphia chromosome (two chromosomes stick together in a way that creates a growth signal that can’t be switched off). The drug Imatinib blocks the action of this broken protein, stalling tumor growth and causing tumor cells to die. Cancers caused by the Philadelphia chromosome are currently very easily treated. Now, these types of drugs don’t exist for every mutation and cancer variation, so DNA testing is required to discover if there is a targeted therapy that works for any given patient. But research into every known mutation and variant is ongoing and new targeted therapy drugs are constantly being developed.
A major disadvantage of seeing cancer as just one disease is that it can hide just how much progress has been made in this field. If cancer is seen as just one disease, then the fact that there are still cases of cancer looks like proof that no progress has been made at all. But by acknowledging this disease’s diversity, we can see just how far we’ve come. Acute promyelocytic leukemia used to have a median survival time of less than a week. Today, up to 90% of patients survive. Medicine is largely moving in a direction that is data driven and highly tailored to the individual, and cancer treatment is a field that will benefit tremendously from this.
I would like to thank Dr. Ancha Baranova, who’s Bio 566 course was where I learned most of this information.
Comments